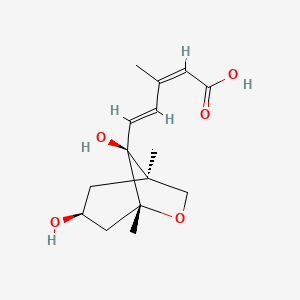
Dihydrophaseic acid
Overview
Description
Dihydrophaseic acid (DPA) is a catabolite of abscisic acid (ABA), a key phytohormone involved in plant stress responses and developmental regulation. DPA is formed via the hydroxylation and isomerization of ABA through the activity of cytochrome P450 enzymes (CYP707A) . Its molecular formula is C₁₅H₂₂O₅ (average mass: 282.336 g/mol), featuring a bicyclic structure with four stereocenters and conjugated double bonds (2Z,4E configuration) . DPA and its derivatives, such as glucosides (e.g., this compound 3'-O-β-gentiobioside), are widely distributed in plants, including Carthamus tinctorius (safflower), Ficus sagittifolia, and Coffea arabica .
Preparation Methods
Biosynthetic Preparation in Plant Tissues
Metabolic Pathways in Plants
Dihydrophaseic acid is synthesized naturally in plants through the oxidative metabolism of ABA. The conversion begins with the hydroxylation of ABA at the 8'-position to form 8'-hydroxyabscisic acid, which spontaneously isomerizes to phaseic acid (PA). Subsequent reduction of PA yields this compound . This pathway is upregulated under environmental stressors such as temperature fluctuations, drought, or salinity, leading to elevated DPA concentrations in plant tissues .
Extraction from Plant Material
The extraction of DPA from plant tissues involves homogenization in ice-cold 90% methanol (10 mL/g fresh tissue), followed by filtration and partitioning into methylene chloride. Acidic fractions containing DPA are derivatized with ethereal diazomethane for gas chromatography (GC) analysis . Key steps include:
-
Homogenization : Disruption of plant cell walls to release metabolites.
-
Solvent Partitioning : Separation of DPA from polar and non-polar contaminants.
-
Derivatization : Enhancement of volatility for GC detection.
Table 1: Optimized Extraction Conditions for DPA from Plant Tissues
Parameter | Condition | Yield (µg/g FW) | Source |
---|---|---|---|
Solvent | 90% methanol | 12.4 ± 1.2 | |
Temperature | 4°C (homogenization) | - | |
Derivatization Agent | Ethereal diazomethane | - | |
Detection Method | GC with electron capture detector | - |
Laboratory Chemical Synthesis
Reduction of Phaseic Acid
Chemical synthesis of DPA involves the selective reduction of the ketone group in phaseic acid. Deuterium-labeled DPA has been synthesized using sodium borodeuteride (NaBD) in aqueous methanol, achieving a deuterium incorporation rate of 86% . The reaction proceeds via nucleophilic attack on the carbonyl carbon, followed by protonation to yield the secondary alcohol.
Table 2: Synthesis Parameters for Deuterium-Labeled DPA
Parameter | Condition | Purity (%) | Source |
---|---|---|---|
Reducing Agent | NaBD (0.1 M) | 86 | |
Solvent | Methanol:Water (9:1) | - | |
Reaction Time | 2 hours | - | |
Purification Method | Sublimation or co-distillation | 95 |
Catalytic Hydrogenation
Alternative methods employ catalytic hydrogenation using palladium on carbon (Pd/C) in tetrahydrofuran (THF). This approach avoids isotopic labeling but requires stringent anhydrous conditions to prevent side reactions .
Extraction and Purification Techniques
Chromatographic Separation
High-performance liquid chromatography (HPLC) with C18 reverse-phase columns is widely used to isolate DPA from complex plant extracts. A gradient elution of acetonitrile and 0.1% formic acid achieves baseline separation of DPA from ABA and PA . Nuclear magnetic resonance (NMR) spectroscopy confirms structural integrity, with characteristic signals at δ 5.72 (H-4) and δ 170.2 (C-1) .
Solvent Recycling in Industrial Processes
Large-scale production may involve solvent recycling to reduce costs. For example, toluene used in diketene dimerization (a side reaction in related syntheses) can be recovered and reused, though residual acetic acid must be removed to maintain yields above 80% .
Analytical Methods for Characterization
Gas Chromatography-Mass Spectrometry (GC-MS)
GC-MS with electron capture detection provides nanogram-level sensitivity for DPA quantification. The method employs a 3% OV-25 column at 225°C, with a retention time of 9.2 minutes for underivatized DPA .
Nuclear Magnetic Resonance (NMR) Spectroscopy
H and C NMR spectra of DPA reveal distinct olefinic protons (δ 5.68–6.12) and a bicyclic ether moiety (δ 78.4–82.1) . ROESY correlations further confirm the stereochemistry of the 6-membered ring .
Challenges and Optimization Strategies
Side Reactions in Chemical Synthesis
The presence of water (>0.1%) during diketene dimerization reduces DPA yields by promoting hydrolysis to acetic acid . Anhydrous conditions and trimethylamine catalysis mitigate this issue, achieving yields up to 96.3% .
Stability Considerations
DPA is prone to epimerization under basic conditions. Storage at -80°C in amber vials minimizes degradation, preserving >90% integrity over six months .
Chemical Reactions Analysis
Types of Reactions: Dihydrophaseic acid undergoes various chemical reactions, including:
Oxidation: It can be oxidized to form phaseic acid.
Reduction: The reduction of phaseic acid to this compound.
Substitution: It can participate in substitution reactions where functional groups are replaced.
Common Reagents and Conditions:
Oxidation: Reagents such as potassium permanganate or chromium trioxide can be used under acidic conditions.
Reduction: Sodium borohydride or lithium aluminum hydride are commonly used reducing agents.
Substitution: Conditions vary depending on the substituent being introduced, but typically involve nucleophilic or electrophilic reagents.
Major Products:
Oxidation: Phaseic acid.
Reduction: this compound.
Substitution: Various substituted derivatives depending on the reagents used.
Scientific Research Applications
Obesity Treatment
Recent studies have identified dihydrophaseic acid glucosides derived from Carthamus tinctorius (safflower) that exhibit anti-adipogenic properties. Specifically, one glucoside variant demonstrated the ability to inhibit lipid accumulation and reduce the expression of adipogenic genes such as Fabp4 and Adipsin in 3T3-L1 preadipocytes. These findings suggest potential therapeutic strategies for obesity management through the modulation of adipocyte differentiation .
Osteoporosis Management
This compound 3'-O-β-d-glucopyranoside has been isolated and studied for its effects on osteoblast differentiation. In vitro experiments revealed that this compound promotes cellular proliferation and mineralized nodule formation in osteoblast lineage cells, indicating its potential as a treatment for osteoporosis . The compound's mechanism involves enhancing alkaline phosphatase activity, which is crucial for bone mineralization.
Plant Stress Responses
As a catabolite of abscisic acid, this compound is involved in regulating plant responses to abiotic stresses such as drought and salinity. It helps maintain ABA homeostasis, which is vital for seed development and stress adaptation. Research has shown that mutations affecting ABA transport can lead to increased levels of this compound, suggesting its role in fine-tuning plant responses under stress conditions .
Comparative Analysis of this compound Applications
Case Study 1: Anti-Adipogenic Effects
A study conducted on this compound glucosides from safflower demonstrated significant anti-adipogenic effects when tested on 3T3-L1 cells. The glucoside variant effectively inhibited lipid droplet accumulation and downregulated key adipogenic markers, showcasing its potential utility in obesity treatment strategies .
Case Study 2: Osteoblast Differentiation
In vitro studies on this compound 3'-O-β-d-glucopyranoside revealed its capacity to enhance osteoblast differentiation significantly. The compound was shown to increase alkaline phosphatase activity, which is an early marker of osteoblast differentiation, indicating its promise as a therapeutic agent for osteoporosis .
Mechanism of Action
Dihydrophaseic acid exerts its effects through various molecular targets and pathways:
Osteoblast Differentiation: It enhances cell proliferation and alkaline phosphatase activity in osteoblasts, leading to increased mineralized nodule formation and expression of osteoblastogenesis markers.
Anti-Adipogenesis: It prevents lipid accumulation in adipocytes by reducing the expression of adipogenic genes.
Anti-Inflammatory Effects: Its stereoisomer has been reported to inhibit tumor necrosis factor-alpha-induced nuclear factor kappa-B activation, suggesting potential anti-inflammatory properties.
Comparison with Similar Compounds
Structural and Functional Comparison with Related Compounds
2.1. Abscisic Acid (ABA)
- Structure: ABA (C₁₅H₂₀O₄) shares a sesquiterpenoid backbone with DPA but lacks the additional hydroxyl group and has a distinct double bond configuration (2Z,4E in DPA vs. 2Z,4E,6E in ABA) .
- Function : ABA is a primary stress hormone regulating stomatal closure, seed dormancy, and drought tolerance. DPA, as an ABA catabolite, exhibits reduced hormonal activity but may modulate ABA signaling through feedback mechanisms .
- Metabolism: ABA is oxidized to phaseic acid (PA) and further reduced to DPA.
2.2. Phaseic Acid (PA)
- Structure : PA (C₁₅H₂₀O₅) is an intermediate in ABA catabolism, differing from DPA by the presence of a ketone group instead of a hydroxyl group at the C-4' position .
- Function : PA weakly mimics ABA in some physiological assays but is less stable than DPA. Both PA and DPA accumulate under stress (e.g., salinity), but DPA is more prevalent in long-term stress adaptation .
2.3. Dihydrophaseic Acid Glucosides
- Structure : DPA glucosides, such as This compound 4-O-β-D-glucoside , are conjugated forms where a glucose moiety enhances solubility and stability .
- Function: Glucosylation likely serves as a detoxification or storage mechanism, analogous to ABA-glucose ester (ABA-GE).
2.4. Other Terpenoid Derivatives
- Unlike DPA, vomifoliol is associated with fruit ripening and lacks ABA-related metabolic roles .
- 3-Acetoxy-8(17),13E-labdadien-15-oic acid: A diterpenoid (C₂₂H₃₂O₄) with anti-inflammatory properties. DPA’s smaller size and ABA-derived structure differentiate its biological roles .
Metabolic and Ecological Roles
- Stress Adaptation: DPA accumulates in roots under nutrient stress, suggesting a role in regulating ABA-mediated root elongation . In carrots, DPA levels rise during cutting-induced stress, correlating with carotenoid biosynthesis .
- Antimicrobial Activity: DPA inhibits P. aeruginosa (MIC: 0.0078 mg/mL) and A. niger (MIC: 0.0039 mg/mL), outperforming reference drugs like gentamycin in some assays .
Biological Activity
Dihydrophaseic acid (DPA) is a significant metabolite derived from abscisic acid (ABA), known for its role in various physiological processes in plants. This article explores the biological activities of DPA, particularly its effects on plant growth, stomatal regulation, and potential therapeutic applications.
Chemical Structure and Metabolism
This compound is a catabolite of phaseic acid (PA), which itself is a product of ABA degradation. The metabolic pathway involves the hydroxylation of ABA, leading to the formation of PA, which is then further reduced to DPA. The chemical structure of DPA can be represented as follows:
This compound exhibits distinct biological activities that are crucial for plant development and stress responses.
1. Stomatal Regulation
Research has shown that DPA does not induce stomatal closure in various plant species, contrasting with ABA and PA. In studies involving Commelina communis, stomatal closure was observed within minutes after treatment with ABA and PA, but DPA failed to elicit a similar response at concentrations up to 10 micromolar across several species, including Zea mays and Hordeum vulgare . This indicates that while DPA is a product of ABA metabolism, it lacks the physiological activity associated with stomatal regulation.
2. Effects on Photosynthesis
DPA has been implicated in reducing photosynthetic efficiency when applied to plant leaves. Studies indicate that treatments with PA and DPA lead to decreased photosynthesis rates, suggesting that these compounds may play a role in regulating plant responses to environmental stressors by modulating photosynthetic activity .
3. Adipogenesis Inhibition
Recent findings have highlighted the potential therapeutic applications of this compound glucosides derived from Carthamus tinctorius. These glucosides have demonstrated significant inhibitory effects on adipogenesis in 3T3-L1 preadipocytes. Specifically, one glucoside prevented lipid droplet accumulation and downregulated adipogenic gene expression, indicating a potential role in obesity treatment . This suggests that derivatives of DPA may possess pharmacological properties beyond their roles in plant physiology.
Comparative Biological Activity
Compound | Stomatal Closure | Photosynthesis Reduction | Adipogenesis Inhibition |
---|---|---|---|
Abscisic Acid | Yes | Yes | Moderate |
Phaseic Acid | Yes | Yes | Low |
This compound | No | Yes | High (in glucoside form) |
Case Studies
Case Study 1: Stomatal Response Analysis
In a study examining the effects of various ABA metabolites on stomatal behavior, it was found that while both ABA and PA effectively closed stomata within four minutes, DPA showed no significant effect across multiple species tested. This highlights the reduced biological activity of DPA compared to its precursors .
Case Study 2: Anti-Adipogenic Effects
A study on this compound glucosides isolated from safflower demonstrated their ability to inhibit adipocyte differentiation significantly. Treatment with these glucosides reduced lipid accumulation and downregulated key adipogenic genes such as Fabp4 and Adipsin, suggesting their potential use in managing obesity .
Q & A
Basic Research Questions
Q. What are the recommended analytical methods for identifying and quantifying dihydrophaseic acid in plant tissues?
- Methodology : Use high-performance liquid chromatography (HPLC) coupled with tandem mass spectrometry (LC-MS/MS) for precise quantification. For structural confirmation, nuclear magnetic resonance (NMR) spectroscopy (¹H and ¹³C) is critical, especially to resolve stereochemical features like the (2Z,4E)-double bond system and bicyclic structure .
- Validation : Include deuterium-labeled internal standards (e.g., deuterated phaseic acid) to correct for matrix effects during extraction .
Q. How should this compound be stored to ensure chemical stability in laboratory settings?
- Storage Protocol : Store DPA as a lyophilized powder at 2–8°C in airtight, light-protected containers. For dissolved samples (e.g., in DMSO), aliquot and store at -20°C to prevent oxidation or hydrolysis .
Q. What are the primary biological sources of this compound, and how is it extracted?
- Extraction Workflow : DPA is a catabolite of abscisic acid (ABA) and is enriched in plant tissues under abiotic stress. Use methanol:water (70:30 v/v) extraction with sonication, followed by solid-phase extraction (SPE) using C18 cartridges to isolate DPA from complex matrices like roots or seeds .
Advanced Research Questions
Q. How can researchers resolve contradictions in DPA accumulation data under water-deficit stress across plant species?
- Experimental Design :
- Controlled Variables : Standardize stress duration, soil water potential (Ψstem), and sampling timepoints (e.g., pre-dawn vs. midday).
- Multi-Omics Integration : Pair metabolomic profiling (LC-MS) with transcriptomic data (e.g., ABA2, ABA3 gene expression) to correlate DPA levels with ABA metabolic flux .
Q. What methodologies are used to study DPA’s role in regulating root growth under nutrient stress?
- Hydroponic Systems : Use flow-controlled hydroponics to modulate solution flow rates, which alter organic acid metabolism. Monitor DPA and N-acetyl-D-mannosamine via spatial metabolomics (MALDI imaging) to map root-zone-specific accumulation .
- Pathway Enrichment : Apply KEGG analysis to identify enriched pathways (e.g., “amino sugar metabolism”) linked to DPA-mediated growth regulation .
Q. How can this compound derivatives (e.g., glucosides) be synthesized and characterized for functional studies?
- Synthetic Approach : Enzymatic glycosylation using UDP-glucosyltransferases (UGTs) to produce 4-O-β-D-glucoside derivatives. Purify products via preparative HPLC and validate structures using HR-ESI-MS and 2D NMR (e.g., HSQC, HMBC) .
- Biological Relevance : Test glucoside derivatives for improved stability or transport in plant vascular systems compared to free DPA .
Q. What experimental strategies address batch-to-batch variability in DPA quantification across laboratories?
- Standardization :
- Use certified reference materials (CRMs) from peer-reviewed suppliers (e.g., ChemFaces, cited in Molecules and Phytomedicine studies) .
- Implement inter-laboratory calibration with spiked recovery tests (e.g., 80–120% recovery range) .
Q. Methodological Resources
- Key Journals : Molecules, Phytochemistry, Plant Physiology (see for full list).
- Critical Techniques :
Note : Avoid non-academic sources (e.g., vendor websites). For synthesis protocols, prioritize methods from Bioscience, Biotechnology, and Biochemistry and Phytochemical Analysis .
Properties
IUPAC Name |
(2Z,4E)-5-[(1R,3S,5R,8S)-3,8-dihydroxy-1,5-dimethyl-6-oxabicyclo[3.2.1]octan-8-yl]-3-methylpenta-2,4-dienoic acid | |
---|---|---|
Source | PubChem | |
URL | https://pubchem.ncbi.nlm.nih.gov | |
Description | Data deposited in or computed by PubChem | |
InChI |
InChI=1S/C15H22O5/c1-10(6-12(17)18)4-5-15(19)13(2)7-11(16)8-14(15,3)20-9-13/h4-6,11,16,19H,7-9H2,1-3H3,(H,17,18)/b5-4+,10-6-/t11-,13+,14+,15-/m0/s1 | |
Source | PubChem | |
URL | https://pubchem.ncbi.nlm.nih.gov | |
Description | Data deposited in or computed by PubChem | |
InChI Key |
XIVFQYWMMJWUCD-VSTJRZLJSA-N | |
Source | PubChem | |
URL | https://pubchem.ncbi.nlm.nih.gov | |
Description | Data deposited in or computed by PubChem | |
Canonical SMILES |
CC(=CC(=O)O)C=CC1(C2(CC(CC1(OC2)C)O)C)O | |
Source | PubChem | |
URL | https://pubchem.ncbi.nlm.nih.gov | |
Description | Data deposited in or computed by PubChem | |
Isomeric SMILES |
C/C(=C/C(=O)O)/C=C/[C@@]1([C@@]2(C[C@@H](C[C@]1(OC2)C)O)C)O | |
Source | PubChem | |
URL | https://pubchem.ncbi.nlm.nih.gov | |
Description | Data deposited in or computed by PubChem | |
Molecular Formula |
C15H22O5 | |
Source | PubChem | |
URL | https://pubchem.ncbi.nlm.nih.gov | |
Description | Data deposited in or computed by PubChem | |
DSSTOX Substance ID |
DTXSID301315742 | |
Record name | Dihydrophaseic acid | |
Source | EPA DSSTox | |
URL | https://comptox.epa.gov/dashboard/DTXSID301315742 | |
Description | DSSTox provides a high quality public chemistry resource for supporting improved predictive toxicology. | |
Molecular Weight |
282.33 g/mol | |
Source | PubChem | |
URL | https://pubchem.ncbi.nlm.nih.gov | |
Description | Data deposited in or computed by PubChem | |
CAS No. |
41756-77-8 | |
Record name | Dihydrophaseic acid | |
Source | CAS Common Chemistry | |
URL | https://commonchemistry.cas.org/detail?cas_rn=41756-77-8 | |
Description | CAS Common Chemistry is an open community resource for accessing chemical information. Nearly 500,000 chemical substances from CAS REGISTRY cover areas of community interest, including common and frequently regulated chemicals, and those relevant to high school and undergraduate chemistry classes. This chemical information, curated by our expert scientists, is provided in alignment with our mission as a division of the American Chemical Society. | |
Explanation | The data from CAS Common Chemistry is provided under a CC-BY-NC 4.0 license, unless otherwise stated. | |
Record name | Dihydrophaseic acid | |
Source | EPA DSSTox | |
URL | https://comptox.epa.gov/dashboard/DTXSID301315742 | |
Description | DSSTox provides a high quality public chemistry resource for supporting improved predictive toxicology. | |
Record name | Dihydrophaseic acid | |
Source | Human Metabolome Database (HMDB) | |
URL | http://www.hmdb.ca/metabolites/HMDB0038660 | |
Description | The Human Metabolome Database (HMDB) is a freely available electronic database containing detailed information about small molecule metabolites found in the human body. | |
Explanation | HMDB is offered to the public as a freely available resource. Use and re-distribution of the data, in whole or in part, for commercial purposes requires explicit permission of the authors and explicit acknowledgment of the source material (HMDB) and the original publication (see the HMDB citing page). We ask that users who download significant portions of the database cite the HMDB paper in any resulting publications. | |
Retrosynthesis Analysis
AI-Powered Synthesis Planning: Our tool employs the Template_relevance Pistachio, Template_relevance Bkms_metabolic, Template_relevance Pistachio_ringbreaker, Template_relevance Reaxys, Template_relevance Reaxys_biocatalysis model, leveraging a vast database of chemical reactions to predict feasible synthetic routes.
One-Step Synthesis Focus: Specifically designed for one-step synthesis, it provides concise and direct routes for your target compounds, streamlining the synthesis process.
Accurate Predictions: Utilizing the extensive PISTACHIO, BKMS_METABOLIC, PISTACHIO_RINGBREAKER, REAXYS, REAXYS_BIOCATALYSIS database, our tool offers high-accuracy predictions, reflecting the latest in chemical research and data.
Strategy Settings
Precursor scoring | Relevance Heuristic |
---|---|
Min. plausibility | 0.01 |
Model | Template_relevance |
Template Set | Pistachio/Bkms_metabolic/Pistachio_ringbreaker/Reaxys/Reaxys_biocatalysis |
Top-N result to add to graph | 6 |
Feasible Synthetic Routes
Disclaimer and Information on In-Vitro Research Products
Please be aware that all articles and product information presented on BenchChem are intended solely for informational purposes. The products available for purchase on BenchChem are specifically designed for in-vitro studies, which are conducted outside of living organisms. In-vitro studies, derived from the Latin term "in glass," involve experiments performed in controlled laboratory settings using cells or tissues. It is important to note that these products are not categorized as medicines or drugs, and they have not received approval from the FDA for the prevention, treatment, or cure of any medical condition, ailment, or disease. We must emphasize that any form of bodily introduction of these products into humans or animals is strictly prohibited by law. It is essential to adhere to these guidelines to ensure compliance with legal and ethical standards in research and experimentation.