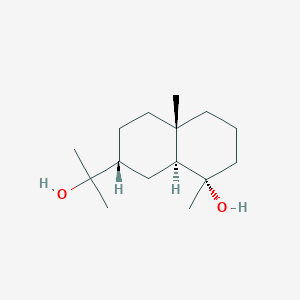
Pterodondiol
Overview
Description
Mechanism of Action
Target of Action
Pterodondiol, a eudesmane-type sesquiterpene compound , primarily targets the Toll-like receptor 7 (TLR7) , myeloid differentiation primary response protein 88 (MyD88) , and tumor necrosis factor (TNF) receptor-associated factor 6 (TRAF6) . These proteins play a crucial role in the immune response to viral infections, particularly influenza A .
Mode of Action
This compound interacts with its targets by inhibiting their expression . Specifically, it inhibits the phosphorylation of p65, a subunit of the NF-κB complex . This inhibition prevents the translocation of p65/NF-κB into the nucleus , thereby suppressing the transcription of genes involved in inflammatory responses .
Biochemical Pathways
The primary biochemical pathway affected by this compound is the TLR7/MyD88/TRAF6/NF-κB signaling pathway . By inhibiting key proteins in this pathway, this compound disrupts the downstream effects, which include the production of pro-inflammatory cytokines such as interleukin (IL)-1β, IL-6, IL-8, and C-C motif chemokine 2 (MCP-1) .
Pharmacokinetics
It’s known that this compound is a metabolite found on the leaves of laggera pterodonta , suggesting that it may be absorbed and metabolized in organisms that consume these leaves.
Result of Action
The primary result of this compound’s action is the inhibition of influenza A virus infection . By suppressing the TLR7/MyD88/TRAF6/NF-κB signaling pathway, this compound prevents the production of pro-inflammatory cytokines . This anti-inflammatory effect can help to mitigate the symptoms of influenza A infection .
Action Environment
The action of this compound may be influenced by various environmental factors. For instance, the concentration of this compound in the environment, which depends on factors such as the growth conditions of Laggera pterodonta , could affect its bioavailability and thus its efficacy.
Biochemical Analysis
Biochemical Properties
Pterodondiol plays a crucial role in various biochemical reactions. It interacts with several enzymes and proteins, influencing their activity. Notably, this compound has been shown to inhibit Toll-like receptor 7 (TLR7), myeloid differentiation primary response protein 88 (MyD88), and tumor necrosis factor receptor-associated factor 6 (TRAF6). These interactions lead to the inhibition of the NF-κB signaling pathway, which is pivotal in inflammatory responses .
Cellular Effects
This compound exerts significant effects on various cell types and cellular processes. It has been demonstrated to inhibit the replication of influenza A virus by modulating the TLR7/MyD88/TRAF6/NF-κB signaling pathway. This modulation results in decreased cytokine production, including interleukin-1β (IL-1β), interleukin-6 (IL-6), and interleukin-8 (IL-8), thereby reducing inflammation . Additionally, this compound influences cell signaling pathways, gene expression, and cellular metabolism, contributing to its antiviral and anti-inflammatory effects.
Molecular Mechanism
The molecular mechanism of this compound involves its interaction with specific biomolecules. This compound binds to TLR7, inhibiting its activation and subsequent signaling cascade. This inhibition prevents the phosphorylation of p65, a subunit of NF-κB, thereby blocking its nuclear translocation and transcriptional activity. Consequently, the expression of pro-inflammatory cytokines is reduced . This compound also interacts with other proteins and enzymes, modulating their activity and contributing to its overall biological effects.
Temporal Effects in Laboratory Settings
In laboratory settings, the effects of this compound have been observed to change over time. Studies have shown that this compound remains stable under various conditions, maintaining its biological activity. Prolonged exposure to this compound can lead to its degradation, resulting in reduced efficacy. Long-term studies have indicated that this compound can exert sustained effects on cellular function, including prolonged inhibition of the NF-κB signaling pathway and reduced cytokine production .
Dosage Effects in Animal Models
The effects of this compound vary with different dosages in animal models. At lower doses, this compound exhibits potent antiviral and anti-inflammatory effects without significant toxicity. At higher doses, this compound can induce adverse effects, including hepatotoxicity and nephrotoxicity. Threshold effects have been observed, where the efficacy of this compound plateaus beyond a certain dosage, indicating the importance of optimizing dosage for therapeutic applications .
Metabolic Pathways
This compound is involved in several metabolic pathways, interacting with various enzymes and cofactors. It has been shown to modulate metabolic flux and alter metabolite levels. This compound’s interaction with enzymes such as cytochrome P450 can influence its metabolism and bioavailability. Additionally, this compound can affect the levels of key metabolites, contributing to its overall biological effects .
Transport and Distribution
This compound is transported and distributed within cells and tissues through specific transporters and binding proteins. It has been observed to accumulate in certain tissues, including the liver and kidneys. This compound’s localization and accumulation can influence its activity and efficacy. Transporters such as ATP-binding cassette (ABC) transporters play a role in the cellular uptake and distribution of this compound .
Subcellular Localization
This compound exhibits specific subcellular localization, which can affect its activity and function. It has been found to localize in the cytoplasm and nucleus of cells. The presence of targeting signals and post-translational modifications can direct this compound to specific compartments or organelles. This subcellular localization is crucial for its interaction with biomolecules and subsequent biological effects .
Preparation Methods
Synthetic Routes and Reaction Conditions
Pterodondiol can be isolated from the leaves of Laggera pterodonta through extraction and purification processes. The isolation involves solvent extraction followed by chromatographic techniques such as high-performance liquid chromatography (HPLC) to achieve high purity .
Industrial Production Methods
Industrial production of this compound is not widely documented, but it typically involves large-scale extraction from plant sources. The process includes harvesting the plant material, drying, and then performing solvent extraction. The extract is then subjected to purification processes to isolate this compound .
Chemical Reactions Analysis
Types of Reactions
Pterodondiol undergoes various chemical reactions, including:
Oxidation: this compound can be oxidized to form corresponding ketones or aldehydes.
Reduction: It can be reduced to form alcohol derivatives.
Substitution: This compound can undergo substitution reactions where functional groups are replaced by other groups.
Common Reagents and Conditions
Oxidation: Common oxidizing agents include potassium permanganate and chromium trioxide.
Reduction: Reducing agents such as sodium borohydride and lithium aluminum hydride are used.
Substitution: Reagents like halogens and nucleophiles are used under various conditions.
Major Products
The major products formed from these reactions include various oxidized and reduced derivatives of this compound, which can be further utilized in different chemical syntheses .
Scientific Research Applications
Pterodondiol has several scientific research applications:
Chemistry: It is used as a precursor in the synthesis of other complex organic molecules.
Medicine: It has been studied for its antiviral properties, particularly against influenza viruses.
Industry: This compound is used in the development of natural product-based pharmaceuticals and agrochemicals.
Comparison with Similar Compounds
Pterodondiol is unique among sesquiterpenes due to its specific biological activities and molecular structure. Similar compounds include:
Pterodontic acid: Another sesquiterpene found in Laggera pterodonta with similar antiviral properties.
Cryptomeridiol: A related sesquiterpene with different biological activities.
This compound stands out due to its specific inhibition of the TLR7/MyD88/NF-κB pathway, which is not commonly observed in other sesquiterpenes .
Properties
IUPAC Name |
(1S,4aS,7R,8aS)-7-(2-hydroxypropan-2-yl)-1,4a-dimethyl-2,3,4,5,6,7,8,8a-octahydronaphthalen-1-ol | |
---|---|---|
Source | PubChem | |
URL | https://pubchem.ncbi.nlm.nih.gov | |
Description | Data deposited in or computed by PubChem | |
InChI |
InChI=1S/C15H28O2/c1-13(2,16)11-6-9-14(3)7-5-8-15(4,17)12(14)10-11/h11-12,16-17H,5-10H2,1-4H3/t11-,12+,14+,15+/m1/s1 | |
Source | PubChem | |
URL | https://pubchem.ncbi.nlm.nih.gov | |
Description | Data deposited in or computed by PubChem | |
InChI Key |
LKKDASYGWYYFIK-DHMWGJHJSA-N | |
Source | PubChem | |
URL | https://pubchem.ncbi.nlm.nih.gov | |
Description | Data deposited in or computed by PubChem | |
Canonical SMILES |
CC12CCCC(C1CC(CC2)C(C)(C)O)(C)O | |
Source | PubChem | |
URL | https://pubchem.ncbi.nlm.nih.gov | |
Description | Data deposited in or computed by PubChem | |
Isomeric SMILES |
C[C@@]12CCC[C@]([C@H]1C[C@@H](CC2)C(C)(C)O)(C)O | |
Source | PubChem | |
URL | https://pubchem.ncbi.nlm.nih.gov | |
Description | Data deposited in or computed by PubChem | |
Molecular Formula |
C15H28O2 | |
Source | PubChem | |
URL | https://pubchem.ncbi.nlm.nih.gov | |
Description | Data deposited in or computed by PubChem | |
Molecular Weight |
240.38 g/mol | |
Source | PubChem | |
URL | https://pubchem.ncbi.nlm.nih.gov | |
Description | Data deposited in or computed by PubChem | |
Retrosynthesis Analysis
AI-Powered Synthesis Planning: Our tool employs the Template_relevance Pistachio, Template_relevance Bkms_metabolic, Template_relevance Pistachio_ringbreaker, Template_relevance Reaxys, Template_relevance Reaxys_biocatalysis model, leveraging a vast database of chemical reactions to predict feasible synthetic routes.
One-Step Synthesis Focus: Specifically designed for one-step synthesis, it provides concise and direct routes for your target compounds, streamlining the synthesis process.
Accurate Predictions: Utilizing the extensive PISTACHIO, BKMS_METABOLIC, PISTACHIO_RINGBREAKER, REAXYS, REAXYS_BIOCATALYSIS database, our tool offers high-accuracy predictions, reflecting the latest in chemical research and data.
Strategy Settings
Precursor scoring | Relevance Heuristic |
---|---|
Min. plausibility | 0.01 |
Model | Template_relevance |
Template Set | Pistachio/Bkms_metabolic/Pistachio_ringbreaker/Reaxys/Reaxys_biocatalysis |
Top-N result to add to graph | 6 |
Feasible Synthetic Routes
Disclaimer and Information on In-Vitro Research Products
Please be aware that all articles and product information presented on BenchChem are intended solely for informational purposes. The products available for purchase on BenchChem are specifically designed for in-vitro studies, which are conducted outside of living organisms. In-vitro studies, derived from the Latin term "in glass," involve experiments performed in controlled laboratory settings using cells or tissues. It is important to note that these products are not categorized as medicines or drugs, and they have not received approval from the FDA for the prevention, treatment, or cure of any medical condition, ailment, or disease. We must emphasize that any form of bodily introduction of these products into humans or animals is strictly prohibited by law. It is essential to adhere to these guidelines to ensure compliance with legal and ethical standards in research and experimentation.