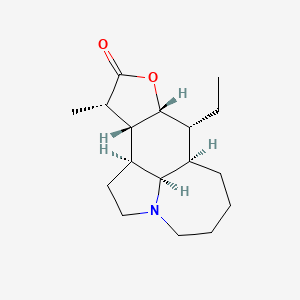
Neostenine
Overview
Description
Neostenine is an organic compound with the molecular formula C17H27NO2 . It is a pale yellow to brown crystal that is soluble in ethanol and chloroform but almost insoluble in water . Neostenine has a wide range of applications in biological and medical research fields. It exhibits various biological activities such as antibacterial, antiviral, antitumor, and antioxidant properties .
Synthesis Analysis
The synthesis of Neostenine has been achieved through a chirality transfer approach using acyclic polyol intermediates . The key step in the synthesis is the sequential Overman/Claisen rearrangement of an allylic 1,2-diol, which introduces two contiguous stereocenters with complete diastereoselectivity in a one-pot sequence .
Molecular Structure Analysis
The molecular structure of Neostenine consists of 17 carbon atoms, 27 hydrogen atoms, and 2 oxygen atoms . The exact mass of Neostenine is 277.204179104 g/mol . The IUPAC name for Neostenine is (1S,9R,10R,11R,14S,15R,16R)-10-ethyl-14-methyl-12-oxa-4-azatetracyclo [7.6.1.04,16.011,15]hexadecan-13-one .
Chemical Reactions Analysis
The key chemical reaction involved in the synthesis of Neostenine is the Diels-Alder/Schmidt reaction . This reaction sequence provides rapid access to the core skeleton shared by several Stemona alkaloids including stenine, neostenine, tuberostemonine, and neotuberostemonine .
Physical And Chemical Properties Analysis
Neostenine has a molecular weight of 277.4 g/mol . It is a pale yellow to brown crystal that is soluble in ethanol and chloroform but almost insoluble in water . The melting point of Neostenine is 90-92°C .
Scientific Research Applications
Synthesis of Neostenine
Neostenine is a Stemona alkaloid . A concise, linear synthesis of Neostenine has been reported . Key features of this synthesis include an organocopper-mediated bislactone C2-desymmetrization for the stereoselective construction of the cyclohexane−lactone C,D-rings . The assembly of the fused pyrrolo [1,2- a ]azepine core was achieved by application of a [5 + 2] maleimide photocycloaddition .
Antitussive Activity
Neostenine has been demonstrated to have significant antitussive activity . This was shown using the citric acid-induced guinea pig cough model .
Insecticidal Activities
Stemona alkaloids, including Neostenine, have shown significant insecticidal activities . These activities have been investigated using biochemical and electrophysiological approaches .
Acetylcholinesterase Inhibitory Properties
Stemona alkaloids have been screened for acetylcholinesterase inhibitory properties . This screening revealed a much higher potency for stemofoline derivatives compared to pyridoazepines, but also showed significant activity for isomers of stenine with a stichoneurine skeleton .
Anti-inflammatory Activity
Some derivatives of Stemona alkaloids have shown anti-inflammatory activity . This activity was identified as an inhibition of the expression of inflammatory mediators .
Reversal of Permeability Glycoprotein-mediated Multidrug Resistance
Evaluating synergistic growth inhibitory effects with cancer chemotherapeutic agents, stemofoline exhibited the most potent effect in the reversal of permeability glycoprotein-mediated multidrug resistance .
Future Directions
The synthesis and study of Neostenine and its derivatives present interesting opportunities for future research. Given its various biological activities, Neostenine could be a potential candidate for drug development and testing . Further studies are needed to elucidate its specific mechanisms of action and potential therapeutic applications.
properties
IUPAC Name |
(1S,9R,10R,11R,14S,15R,16R)-10-ethyl-14-methyl-12-oxa-4-azatetracyclo[7.6.1.04,16.011,15]hexadecan-13-one | |
---|---|---|
Source | PubChem | |
URL | https://pubchem.ncbi.nlm.nih.gov | |
Description | Data deposited in or computed by PubChem | |
InChI |
InChI=1S/C17H27NO2/c1-3-11-12-6-4-5-8-18-9-7-13(15(12)18)14-10(2)17(19)20-16(11)14/h10-16H,3-9H2,1-2H3/t10-,11+,12+,13-,14-,15+,16+/m0/s1 | |
Source | PubChem | |
URL | https://pubchem.ncbi.nlm.nih.gov | |
Description | Data deposited in or computed by PubChem | |
InChI Key |
ROIHYOJMCBKEER-FGBMJVKNSA-N | |
Source | PubChem | |
URL | https://pubchem.ncbi.nlm.nih.gov | |
Description | Data deposited in or computed by PubChem | |
Canonical SMILES |
CCC1C2CCCCN3C2C(CC3)C4C1OC(=O)C4C | |
Source | PubChem | |
URL | https://pubchem.ncbi.nlm.nih.gov | |
Description | Data deposited in or computed by PubChem | |
Isomeric SMILES |
CC[C@@H]1[C@H]2CCCCN3[C@H]2[C@@H](CC3)[C@H]4[C@@H]1OC(=O)[C@H]4C | |
Source | PubChem | |
URL | https://pubchem.ncbi.nlm.nih.gov | |
Description | Data deposited in or computed by PubChem | |
Molecular Formula |
C17H27NO2 | |
Source | PubChem | |
URL | https://pubchem.ncbi.nlm.nih.gov | |
Description | Data deposited in or computed by PubChem | |
Molecular Weight |
277.4 g/mol | |
Source | PubChem | |
URL | https://pubchem.ncbi.nlm.nih.gov | |
Description | Data deposited in or computed by PubChem | |
Product Name |
Neostenine |
Retrosynthesis Analysis
AI-Powered Synthesis Planning: Our tool employs the Template_relevance Pistachio, Template_relevance Bkms_metabolic, Template_relevance Pistachio_ringbreaker, Template_relevance Reaxys, Template_relevance Reaxys_biocatalysis model, leveraging a vast database of chemical reactions to predict feasible synthetic routes.
One-Step Synthesis Focus: Specifically designed for one-step synthesis, it provides concise and direct routes for your target compounds, streamlining the synthesis process.
Accurate Predictions: Utilizing the extensive PISTACHIO, BKMS_METABOLIC, PISTACHIO_RINGBREAKER, REAXYS, REAXYS_BIOCATALYSIS database, our tool offers high-accuracy predictions, reflecting the latest in chemical research and data.
Strategy Settings
Precursor scoring | Relevance Heuristic |
---|---|
Min. plausibility | 0.01 |
Model | Template_relevance |
Template Set | Pistachio/Bkms_metabolic/Pistachio_ringbreaker/Reaxys/Reaxys_biocatalysis |
Top-N result to add to graph | 6 |
Feasible Synthetic Routes
Q & A
Q1: What is the primary biological activity reported for Neostenine?
A1: Neostenine has shown significant antitussive activity in preclinical studies using guinea pig models. [] Specifically, it demonstrated efficacy in reducing cough frequency induced by citric acid aerosol stimulation. []
Q2: How does the structure of Neostenine relate to its antitussive activity?
A2: Research suggests that the saturated tricyclic pyrrolo[3,2,1-jk]benzazepine nucleus within the Neostenine structure is crucial for its antitussive activity. [] Furthermore, the all-cis configuration at the three ring junctions appears to be the optimal configuration for this specific activity. []
Q3: Have any studies investigated the absorption of Neostenine?
A3: Yes, research using a Caco-2 monolayer model, which mimics the intestinal lining, indicates Neostenine exhibits high absorptive permeability. [] This suggests it is likely well-absorbed following oral administration. []
Q4: Does Neostenine interact with any transporter proteins?
A4: Studies suggest Neostenine is a substrate for P-glycoprotein (P-gp), an efflux transporter found in the intestine. [] This interaction could potentially influence its absorption and distribution within the body. []
Q5: Are there any other reported biological activities for Neostenine?
A5: Beyond its antitussive effects, Neostenine has shown potential in other areas. It has demonstrated inhibitory activity against lipopolysaccharide-induced nitric oxide production in murine BV2 microglial cells, suggesting possible anti-inflammatory properties. [] Additionally, research into synthetic Stemona alkaloid analogues, inspired by natural products like Neostenine, has revealed a class of potent sigma ligands. [] These findings highlight the potential of Neostenine and its analogues as valuable tools for exploring various biological pathways.
Q6: What synthetic strategies have been explored to produce Neostenine?
A6: Several total syntheses of Neostenine have been achieved, showcasing the evolution of synthetic organic chemistry. One approach utilized a chirality transfer strategy with acyclic polyol intermediates, culminating in the first enantioselective total synthesis. [] Another elegant approach employed a protecting group-free synthesis featuring a [5+2] maleimide photocycloaddition to assemble the fused pyrrolo[1,2-a]azepine core, highlighting a concise and efficient route. []
Q7: Are there any known sources of Neostenine besides laboratory synthesis?
A7: Yes, Neostenine is a naturally occurring alkaloid found in the roots of Stemona tuberosa, a plant used in traditional Chinese medicine. [, ]
Q8: Have any computational studies been conducted on Neostenine or its analogues?
A8: While the provided abstracts don't explicitly detail computational studies on Neostenine itself, research on Stemona alkaloid analogues involved screening over 100 analogues for activity against G protein-coupled receptors. [] This suggests computational methods might have been employed for initial screening or to guide analogue design.
Disclaimer and Information on In-Vitro Research Products
Please be aware that all articles and product information presented on BenchChem are intended solely for informational purposes. The products available for purchase on BenchChem are specifically designed for in-vitro studies, which are conducted outside of living organisms. In-vitro studies, derived from the Latin term "in glass," involve experiments performed in controlled laboratory settings using cells or tissues. It is important to note that these products are not categorized as medicines or drugs, and they have not received approval from the FDA for the prevention, treatment, or cure of any medical condition, ailment, or disease. We must emphasize that any form of bodily introduction of these products into humans or animals is strictly prohibited by law. It is essential to adhere to these guidelines to ensure compliance with legal and ethical standards in research and experimentation.