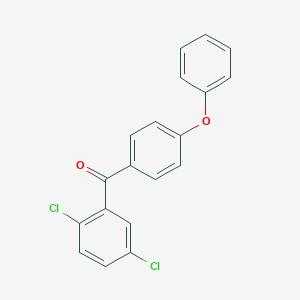
2,5-Dichloro-4'-phenoxybenzophenone
Overview
Description
2,5-Dichloro-4’-phenoxybenzophenone is an organic compound with the molecular formula C19H12Cl2O2 and a molecular weight of 343.20 g/mol . It is characterized by the presence of two chlorine atoms and a phenoxy group attached to a benzophenone core. This compound is typically found as white to pale yellow crystals or crystalline powder .
Preparation Methods
Synthetic Routes and Reaction Conditions
The synthesis of 2,5-Dichloro-4’-phenoxybenzophenone can be achieved through various methods. One common approach involves the Friedel-Crafts acylation reaction, where 2,5-dichlorobenzoyl chloride reacts with phenol in the presence of a Lewis acid catalyst such as aluminum chloride (AlCl3). The reaction is typically carried out under anhydrous conditions and requires careful control of temperature and reaction time to achieve high yields .
Industrial Production Methods
In industrial settings, the production of 2,5-Dichloro-4’-phenoxybenzophenone may involve large-scale batch or continuous processes. The reaction conditions are optimized to ensure maximum efficiency and purity of the final product. The use of advanced purification techniques, such as recrystallization and chromatography, helps in obtaining the compound with high purity suitable for various applications .
Chemical Reactions Analysis
Types of Reactions
2,5-Dichloro-4’-phenoxybenzophenone undergoes several types of chemical reactions, including:
Substitution Reactions: The chlorine atoms in the compound can be substituted by other nucleophiles, such as amines or thiols, under appropriate conditions.
Oxidation and Reduction: The compound can undergo oxidation to form quinones or reduction to form hydroquinones, depending on the reagents and conditions used.
Coupling Reactions: It can participate in coupling reactions, such as Suzuki or Heck coupling, to form more complex aromatic structures.
Common Reagents and Conditions
Substitution Reactions: Common reagents include nucleophiles like sodium methoxide or potassium tert-butoxide, often in polar aprotic solvents like dimethyl sulfoxide (DMSO).
Oxidation: Reagents such as potassium permanganate (KMnO4) or chromium trioxide (CrO3) in acidic or basic media.
Major Products
The major products formed from these reactions depend on the specific conditions and reagents used. For example, substitution reactions can yield various substituted benzophenones, while oxidation can produce quinones .
Scientific Research Applications
2,5-Dichloro-4’-phenoxybenzophenone has a wide range of applications in scientific research:
Chemistry: It is used as an intermediate in the synthesis of various organic compounds, including pharmaceuticals and agrochemicals.
Biology: The compound is studied for its potential biological activities, such as antimicrobial and anticancer properties.
Medicine: Research is ongoing to explore its potential as a therapeutic agent in treating various diseases.
Industry: It is used in the production of specialty chemicals, dyes, and polymers.
Mechanism of Action
The mechanism of action of 2,5-Dichloro-4’-phenoxybenzophenone involves its interaction with specific molecular targets and pathways. The compound can act as an inhibitor or modulator of certain enzymes or receptors, leading to changes in cellular processes. For example, it may inhibit the activity of enzymes involved in cell proliferation, thereby exhibiting anticancer properties .
Comparison with Similar Compounds
Similar Compounds
2,4-Dichlorobenzophenone: Similar in structure but with chlorine atoms at different positions.
4,4’-Dichlorobenzophenone: Both chlorine atoms are on the same benzene ring.
2,5-Dichlorobenzophenone: Lacks the phenoxy group present in 2,5-Dichloro-4’-phenoxybenzophenone.
Uniqueness
2,5-Dichloro-4’-phenoxybenzophenone is unique due to the presence of both chlorine atoms and a phenoxy group, which imparts distinct chemical and biological properties. This combination allows for specific interactions with molecular targets, making it valuable in various research and industrial applications .
Properties
IUPAC Name |
(2,5-dichlorophenyl)-(4-phenoxyphenyl)methanone | |
---|---|---|
Source | PubChem | |
URL | https://pubchem.ncbi.nlm.nih.gov | |
Description | Data deposited in or computed by PubChem | |
InChI |
InChI=1S/C19H12Cl2O2/c20-14-8-11-18(21)17(12-14)19(22)13-6-9-16(10-7-13)23-15-4-2-1-3-5-15/h1-12H | |
Source | PubChem | |
URL | https://pubchem.ncbi.nlm.nih.gov | |
Description | Data deposited in or computed by PubChem | |
InChI Key |
VHXNNVZZGMWSBN-UHFFFAOYSA-N | |
Source | PubChem | |
URL | https://pubchem.ncbi.nlm.nih.gov | |
Description | Data deposited in or computed by PubChem | |
Canonical SMILES |
C1=CC=C(C=C1)OC2=CC=C(C=C2)C(=O)C3=C(C=CC(=C3)Cl)Cl | |
Source | PubChem | |
URL | https://pubchem.ncbi.nlm.nih.gov | |
Description | Data deposited in or computed by PubChem | |
Molecular Formula |
C19H12Cl2O2 | |
Source | PubChem | |
URL | https://pubchem.ncbi.nlm.nih.gov | |
Description | Data deposited in or computed by PubChem | |
DSSTOX Substance ID |
DTXSID90477222 | |
Record name | 2,5-Dichloro-4'-phenoxybenzophenone | |
Source | EPA DSSTox | |
URL | https://comptox.epa.gov/dashboard/DTXSID90477222 | |
Description | DSSTox provides a high quality public chemistry resource for supporting improved predictive toxicology. | |
Molecular Weight |
343.2 g/mol | |
Source | PubChem | |
URL | https://pubchem.ncbi.nlm.nih.gov | |
Description | Data deposited in or computed by PubChem | |
CAS No. |
151173-25-0 | |
Record name | 2,5-Dichloro-4'-phenoxybenzophenone | |
Source | EPA DSSTox | |
URL | https://comptox.epa.gov/dashboard/DTXSID90477222 | |
Description | DSSTox provides a high quality public chemistry resource for supporting improved predictive toxicology. | |
Synthesis routes and methods I
Procedure details
Synthesis routes and methods II
Procedure details
Retrosynthesis Analysis
AI-Powered Synthesis Planning: Our tool employs the Template_relevance Pistachio, Template_relevance Bkms_metabolic, Template_relevance Pistachio_ringbreaker, Template_relevance Reaxys, Template_relevance Reaxys_biocatalysis model, leveraging a vast database of chemical reactions to predict feasible synthetic routes.
One-Step Synthesis Focus: Specifically designed for one-step synthesis, it provides concise and direct routes for your target compounds, streamlining the synthesis process.
Accurate Predictions: Utilizing the extensive PISTACHIO, BKMS_METABOLIC, PISTACHIO_RINGBREAKER, REAXYS, REAXYS_BIOCATALYSIS database, our tool offers high-accuracy predictions, reflecting the latest in chemical research and data.
Strategy Settings
Precursor scoring | Relevance Heuristic |
---|---|
Min. plausibility | 0.01 |
Model | Template_relevance |
Template Set | Pistachio/Bkms_metabolic/Pistachio_ringbreaker/Reaxys/Reaxys_biocatalysis |
Top-N result to add to graph | 6 |
Feasible Synthetic Routes
Disclaimer and Information on In-Vitro Research Products
Please be aware that all articles and product information presented on BenchChem are intended solely for informational purposes. The products available for purchase on BenchChem are specifically designed for in-vitro studies, which are conducted outside of living organisms. In-vitro studies, derived from the Latin term "in glass," involve experiments performed in controlled laboratory settings using cells or tissues. It is important to note that these products are not categorized as medicines or drugs, and they have not received approval from the FDA for the prevention, treatment, or cure of any medical condition, ailment, or disease. We must emphasize that any form of bodily introduction of these products into humans or animals is strictly prohibited by law. It is essential to adhere to these guidelines to ensure compliance with legal and ethical standards in research and experimentation.