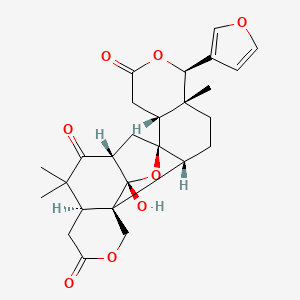
Seneganolide
Overview
Description
Mechanism of Action
- Seneganolide primarily targets the large intestine and is used as a laxative to treat constipation .
- Rheinanthrone appears to increase cyclooxygenase 2 (COX2) expression in macrophage cells, leading to an increase in prostaglandin E2 (PGE2) .
- It stimulates peristaltic activity in the intestine by directly affecting the intestinal mucosa or nerve plexus, thereby increasing motility .
- PGE2 is involved in inflammation and smooth muscle contraction, which contributes to increased intestinal motility and defecation .
Target of Action
Mode of Action
Biochemical Pathways
Pharmacokinetics
Biochemical Analysis
Biochemical Properties
Seneganolide plays a significant role in biochemical reactions, particularly in its interaction with various enzymes and proteins. It has been identified as an insect antifeedant, which suggests its interaction with insect digestive enzymes and receptors. The compound’s structure allows it to bind to specific sites on these enzymes, inhibiting their activity and thus deterring insects from feeding . Additionally, this compound’s interaction with proteins involved in cellular signaling pathways may contribute to its antifeedant properties.
Cellular Effects
This compound influences various cellular processes, including cell signaling pathways, gene expression, and cellular metabolism. In insect cells, this compound disrupts normal cellular functions by inhibiting key enzymes involved in digestion and nutrient absorption. This disruption leads to altered gene expression and metabolic imbalances, ultimately affecting the insect’s ability to thrive . Furthermore, this compound’s impact on cell signaling pathways may extend to other cell types, potentially influencing cellular responses to environmental stimuli.
Molecular Mechanism
At the molecular level, this compound exerts its effects through specific binding interactions with biomolecules. The compound’s structure allows it to fit into the active sites of enzymes, inhibiting their catalytic activity. This inhibition can lead to a cascade of effects, including changes in gene expression and metabolic pathways. This compound’s ability to bind to and inhibit enzymes involved in digestion is a key aspect of its antifeedant properties . Additionally, the compound may interact with receptors on cell membranes, influencing signal transduction pathways and cellular responses.
Temporal Effects in Laboratory Settings
In laboratory settings, the effects of this compound have been observed to change over time. The compound’s stability and degradation play crucial roles in its long-term effects on cellular function. Studies have shown that this compound remains stable under certain conditions, maintaining its inhibitory effects on enzymes and cellular processes . Prolonged exposure to environmental factors such as light and temperature may lead to degradation, reducing its efficacy. Long-term studies in vitro and in vivo have demonstrated that this compound’s effects on cellular function can persist, although the extent of these effects may diminish over time.
Dosage Effects in Animal Models
The effects of this compound vary with different dosages in animal models. At lower doses, the compound effectively inhibits enzyme activity and disrupts cellular processes without causing significant toxicity . At higher doses, this compound may exhibit toxic effects, including cellular damage and metabolic imbalances. Threshold effects have been observed, where a certain dosage is required to achieve the desired inhibitory effects, while exceeding this threshold can lead to adverse outcomes. These findings highlight the importance of dosage optimization in utilizing this compound for its intended applications.
Metabolic Pathways
This compound is involved in various metabolic pathways, interacting with enzymes and cofactors essential for its activity. The compound’s metabolism involves its conversion into active metabolites that retain its inhibitory properties . These metabolites may further interact with enzymes involved in digestion and nutrient absorption, enhancing this compound’s antifeedant effects. Additionally, this compound’s impact on metabolic flux and metabolite levels can influence overall cellular metabolism, contributing to its biochemical properties.
Transport and Distribution
Within cells and tissues, this compound is transported and distributed through specific mechanisms. The compound may interact with transporters and binding proteins that facilitate its movement across cell membranes . Once inside the cell, this compound can localize to specific compartments, such as the cytoplasm or organelles, where it exerts its inhibitory effects. The distribution of this compound within tissues can also influence its overall efficacy, with higher concentrations in target tissues leading to more pronounced effects.
Subcellular Localization
This compound’s subcellular localization plays a crucial role in its activity and function. The compound may be directed to specific cellular compartments through targeting signals or post-translational modifications . For example, this compound may localize to the endoplasmic reticulum or mitochondria, where it can interact with enzymes and proteins involved in metabolic processes. This localization enhances this compound’s ability to inhibit enzyme activity and disrupt cellular functions, contributing to its overall biochemical properties.
Preparation Methods
Synthetic Routes and Reaction Conditions: Seneganolide is typically obtained through extraction from the roots or bark of the Senegalia senegal plant . The extraction process often involves the use of organic solvents such as ethanol or chloroform . The compound can also be synthesized in the laboratory, although detailed synthetic routes and reaction conditions are not extensively documented in the literature.
Industrial Production Methods: Industrial production of this compound primarily relies on the extraction from natural sources. The process involves harvesting the plant material, followed by solvent extraction and purification to isolate the compound. The use of advanced chromatographic techniques ensures the purity and quality of the final product.
Chemical Reactions Analysis
Types of Reactions: Seneganolide undergoes various chemical reactions, including oxidation, reduction, and substitution reactions. These reactions are essential for modifying the compound’s structure and enhancing its properties for specific applications.
Common Reagents and Conditions:
Oxidation: Common oxidizing agents such as potassium permanganate or chromium trioxide can be used to oxidize this compound.
Reduction: Reducing agents like sodium borohydride or lithium aluminum hydride are employed to reduce this compound.
Substitution: Nucleophilic substitution reactions can be carried out using reagents like sodium methoxide or potassium tert-butoxide.
Major Products Formed: The major products formed from these reactions depend on the specific conditions and reagents used. For instance, oxidation may yield various oxidized derivatives, while reduction can produce reduced forms of this compound with altered functional groups.
Scientific Research Applications
Seneganolide has a wide range of scientific research applications, including:
Chemistry: It is used as a model compound for studying limonoid structures and their reactivity.
Industry: The compound is used in the formulation of natural insect repellents and other biopesticides.
Comparison with Similar Compounds
Seneganolide is unique among limonoids due to its specific structure and potent antifeedant properties. Similar compounds include:
3-O-methyl-butyrylthis compound A: Another limonoid isolated from Khaya ivorensis with similar biological activities.
1,3-dideacetylkhivorin: A limonoid with cytotoxic properties against various cancer cell lines.
7-deacetylkhivorin: Known for its antimalarial and anti-inflammatory activities.
These compounds share structural similarities with this compound but differ in their specific biological activities and applications.
Properties
CAS No. |
301530-12-1 |
---|---|
Molecular Formula |
C26H30O8 |
Molecular Weight |
470.5 g/mol |
IUPAC Name |
(1S,2R,6R,7R,10R,11S,16S,19S)-6-(furan-3-yl)-20-hydroxy-7,17,17-trimethyl-5,13,21-trioxahexacyclo[17.2.1.01,10.02,7.011,16.011,20]docosane-4,14,18-trione |
InChI |
InChI=1S/C26H30O8/c1-22(2)16-8-18(27)32-12-24(16)15-4-6-23(3)17(9-19(28)33-21(23)13-5-7-31-11-13)25(15)10-14(20(22)29)26(24,30)34-25/h5,7,11,14-17,21,30H,4,6,8-10,12H2,1-3H3/t14-,15+,16-,17+,21-,23+,24+,25+,26?/m0/s1 |
InChI Key |
VIOKSDWKSSMHBF-MNGWOIGJSA-N |
SMILES |
CC1(C2CC(=O)OCC23C4CCC5(C(C46CC(C1=O)C3(O6)O)CC(=O)OC5C7=COC=C7)C)C |
Isomeric SMILES |
C[C@@]12CC[C@H]3[C@@]4([C@@H]1CC(=O)O[C@H]2C5=COC=C5)C[C@H]6C(=O)C([C@H]7[C@@]3(C6(O4)O)COC(=O)C7)(C)C |
Canonical SMILES |
CC1(C2CC(=O)OCC23C4CCC5(C(C46CC(C1=O)C3(O6)O)CC(=O)OC5C7=COC=C7)C)C |
Appearance |
Powder |
Origin of Product |
United States |
Retrosynthesis Analysis
AI-Powered Synthesis Planning: Our tool employs the Template_relevance Pistachio, Template_relevance Bkms_metabolic, Template_relevance Pistachio_ringbreaker, Template_relevance Reaxys, Template_relevance Reaxys_biocatalysis model, leveraging a vast database of chemical reactions to predict feasible synthetic routes.
One-Step Synthesis Focus: Specifically designed for one-step synthesis, it provides concise and direct routes for your target compounds, streamlining the synthesis process.
Accurate Predictions: Utilizing the extensive PISTACHIO, BKMS_METABOLIC, PISTACHIO_RINGBREAKER, REAXYS, REAXYS_BIOCATALYSIS database, our tool offers high-accuracy predictions, reflecting the latest in chemical research and data.
Strategy Settings
Precursor scoring | Relevance Heuristic |
---|---|
Min. plausibility | 0.01 |
Model | Template_relevance |
Template Set | Pistachio/Bkms_metabolic/Pistachio_ringbreaker/Reaxys/Reaxys_biocatalysis |
Top-N result to add to graph | 6 |
Feasible Synthetic Routes
Q1: What is Seneganolide and where is it found?
A1: this compound is a naturally occurring limonoid, specifically classified as a mexicanolide, known for its insect antifeedant properties. [, ] It was first isolated from the stem bark of the Khaya senegalensis tree, also known as African mahogany. [] This tree species is predominantly found in African countries. [] Later, this compound was also isolated from the fruits of Khaya senegalensis growing in Vietnam. []
Q2: How does this compound exhibit its insect antifeedant activity?
A2: While the exact mechanism of action of this compound remains to be fully elucidated, its antifeedant activity was demonstrated through a conventional leaf disk method. [] This suggests that this compound likely interacts with the insect's chemoreceptors, deterring them from feeding on treated plant material. Further research is needed to understand the specific molecular targets and downstream effects of this compound in insects.
Q3: What is the chemical structure of this compound?
A3: this compound is a unique mexicanolide with a rings B,D-seco limonoid structure. [] Unfortunately, the specific molecular formula and weight are not provided in the given abstracts. To determine the spectroscopic data, one would need to refer to the full research articles.
Q4: Besides its antifeedant activity, has this compound been investigated for other biological activities?
A4: Interestingly, while initially identified as an antifeedant, this compound's co-isolation with other limonoids possessing cytotoxic activities [] hints at its potential for broader biological activity. Further research is necessary to explore these possibilities and uncover potential applications in other fields.
Disclaimer and Information on In-Vitro Research Products
Please be aware that all articles and product information presented on BenchChem are intended solely for informational purposes. The products available for purchase on BenchChem are specifically designed for in-vitro studies, which are conducted outside of living organisms. In-vitro studies, derived from the Latin term "in glass," involve experiments performed in controlled laboratory settings using cells or tissues. It is important to note that these products are not categorized as medicines or drugs, and they have not received approval from the FDA for the prevention, treatment, or cure of any medical condition, ailment, or disease. We must emphasize that any form of bodily introduction of these products into humans or animals is strictly prohibited by law. It is essential to adhere to these guidelines to ensure compliance with legal and ethical standards in research and experimentation.