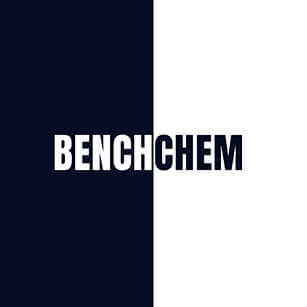
L-Citrulline-d7
- Click on QUICK INQUIRY to receive a quote from our team of experts.
- With the quality product at a COMPETITIVE price, you can focus more on your research.
Description
L-Citrulline-d7, also known as this compound, is a useful research compound. Its molecular formula is C₆H₆D₇N₃O₃ and its molecular weight is 182.23. The purity is usually 95%.
BenchChem offers high-quality this compound suitable for many research applications. Different packaging options are available to accommodate customers' requirements. Please inquire for more information about this compound including the price, delivery time, and more detailed information at info@benchchem.com.
Q & A
Basic Research Questions
Q. What methodological steps ensure the accurate use of L-Citrulline-d7 as an internal standard in quantitative mass spectrometry?
this compound is integrated into workflows to correct for technical variability during sample preparation and instrument analysis. Key steps include:
- Spike-in calibration : Adding a known concentration of this compound to biological samples before extraction to normalize recovery rates.
- MRM optimization : Using transitions like m/z 183.15→166.05 (for this compound) with collision energy (10 V) and cone voltage (15 V) to maximize signal specificity .
- Validation : Performing spike-and-recovery experiments (e.g., 80–120% recovery rates) and cross-validation with unlabeled standards to confirm linearity (R² > 0.99) .
Q. Which analytical techniques are critical for verifying the isotopic purity and chemical stability of this compound?
- High-Resolution Mass Spectrometry (HRMS) : Confirms isotopic enrichment (>98% deuterium incorporation) and detects impurities (e.g., unlabeled citrulline).
- Nuclear Magnetic Resonance (NMR) : Validates structural integrity by analyzing deuterium placement (e.g., δ 3.1–3.5 ppm for methylene groups) .
- HPLC-UV/FLD : Assesses chemical stability under storage conditions (e.g., -80°C in inert atmospheres to prevent degradation) .
Q. How should researchers design pilot studies to establish baseline citrulline levels when introducing this compound in human plasma assays?
- Population sampling : Collect plasma from ≥20 healthy donors to account for biological variability.
- Pre-analytical controls : Standardize fasting periods, centrifugation speeds, and anticoagulants (e.g., EDTA vs. heparin).
- Data normalization : Use this compound/internal standard ratios to correct for matrix effects .
Advanced Research Questions
Q. What strategies mitigate isotopic interference when co-analyzing this compound with endogenous metabolites in complex biological matrices?
- Chromatographic separation : Optimize gradient elution (e.g., 5–95% acetonitrile over 15 min) to resolve this compound from isobaric compounds like arginine-d4.
- Dynamic MRM : Monitor secondary transitions (e.g., m/z 183.15→142.10) to confirm peak identity .
- Mathematical correction : Apply post-acquisition algorithms (e.g., natural abundance correction) to subtract background signals from non-deuterated species.
Q. How can researchers integrate this compound tracer studies with multi-omics workflows to map nitric oxide (NO) metabolism in endothelial cells?
- Experimental design :
- PICOT framework : Population (HUVECs under hypoxia), Intervention (this compound supplementation), Comparison (untreated cells), Outcome (NO flux via LC-MS/MS), Time (0–24h) .
- Multi-omics integration : Pair LC-MS/MS (quantifying this compound incorporation) with RNA-seq to link NO production to endothelial gene networks .
- Data analysis : Use pathway tools (e.g., MetaboAnalyst, KEGG) to overlay isotopic tracing data with proteomic/transcriptomic datasets.
Q. What statistical approaches address batch effects in large-scale studies using this compound across multiple laboratories?
- Preprocessing : Apply ComBat or SVA to normalize inter-lab instrument variability .
- Quality controls : Include pooled reference samples in each batch to monitor precision (%CV < 15%) .
- Mixed-effects models : Account for random effects (e.g., lab-to-lab differences) while testing fixed effects (e.g., treatment response) .
Q. Methodological Challenges & Solutions
Q. How do researchers reconcile discrepancies in this compound pharmacokinetic data between in vivo and ex vivo models?
- Contradiction analysis : Compare hepatic clearance rates in ex vivo liver slices (e.g., 2.3 ± 0.5 mL/min/kg) vs. whole-organism studies, adjusting for perfusion limitations .
- Mechanistic modeling : Use PBPK simulations to extrapolate ex vivo results to in vivo contexts, incorporating tissue-specific binding rates .
Q. What ethical and technical considerations apply when administering this compound in clinical trials involving vulnerable populations (e.g., renal impairment)?
- Ethical compliance : Obtain IRB approval with explicit disclosure of deuterated compound use and long-term safety monitoring .
- Dose adjustment : Reduce this compound doses by 50% in renal-impaired cohorts to avoid accumulation, validated via GFR-adjusted pharmacokinetic models .
Q. Data Presentation Example
Parameter | This compound Value | Unlabeled Citrulline | Method Reference |
---|---|---|---|
MRM Transition (m/z) | 183.15→166.05 | 176.15→159.05 | |
Collision Energy (V) | 10 | 25 | |
Plasma Recovery (%) | 95 ± 5 | N/A | |
Isotopic Purity | >98% | N/A |
Preparation Methods
Isotopic Labeling Strategies for Deuterium Incorporation
Deuterium labeling of L-citrulline introduces seven deuterium atoms into the molecular structure, typically at positions adjacent to nitrogen or oxygen atoms to ensure metabolic stability. Two primary approaches dominate industrial and laboratory settings:
Chemical Synthesis with Deuterated Precursors
Deuterium can be incorporated via chemical synthesis using deuterated reagents. For example, L-ornithine monohydrochloride treated with deuterium oxide (D2O) under alkaline conditions facilitates H/D exchange at labile hydrogen sites . Subsequent formylation of the δ-amino group with deuterated carbamide (CO(ND2)2) ensures further deuteration. Copper salts are often employed to protect the α-amino group during synthesis, though their removal requires careful treatment with sulfides or organic acids to avoid isotopic scrambling .
Table 1: Key Reaction Conditions for Deuteration via Chemical Synthesis
Step | Reagents | Temperature | Duration | Yield (%) |
---|---|---|---|---|
H/D Exchange | D2O, NaOH | 80°C | 24 h | 65–70 |
δ-Amino Formylation | CO(ND2)2, CuSO4 | 50°C | 12 h | 85–90 |
Copper Removal | Na2S, HCl | RT | 2 h | 95 |
Biosynthetic Incorporation Using Deuterated Media
Microbial fermentation in deuterium-enriched media offers an alternative route. Streptococcus faecalis strains cultivated in D2O-based media with deuterated arginine precursors produce this compound through the arginine deiminase pathway . However, microbial tolerance to D2O is limited, requiring gradual adaptation to prevent osmotic stress. Typical deuterium incorporation rates range from 70–80%, with residual protiated species removed via chromatographic purification .
Chemical Synthesis Routes and Optimization
Protection-Formylation-Deprotection Sequence
The synthesis begins with L-ornithine, where the α-amino group is protected using copper(II) sulfate under alkaline conditions (pH 10–12). The δ-amino group is then formylated with carbamide, followed by acid hydrolysis to yield L-citrulline. For deuterated variants, D2O replaces H2O in hydrolysis, ensuring deuteration at exchangeable sites .
Critical Challenges :
-
Copper removal via sulfide treatment generates hazardous waste (e.g., H2S) .
-
Racemization during hydrolysis reduces enantiomeric purity, necessitating low-temperature (≤40°C) conditions .
Direct Deuterium Exchange Post-Synthesis
Post-synthetic deuteration involves treating L-citrulline with D2O at elevated temperatures (80–100°C) in the presence of palladium or platinum catalysts. This method achieves 90–95% deuteration at labile hydrogens but fails to label non-exchangeable positions, limiting its utility for full deuteration .
Industrial-Scale Biotechnological Production
Microbial Conversion of Deuterated Arginine
Industrial processes leverage Streptococcus faecalis to convert deuterated L-arginine into this compound. The arginine deiminase pathway catalyzes the reaction:
2\text{O} \rightarrow \text{this compound} + \text{NH}3 L-arginine+D2O→This compound+NH3
Fermentation Parameters :
-
pH: 6.0–6.5 (buffered with sodium dodecyl sulfate)
-
Temperature: 37°C
-
Agitation: 300 rpm
Table 2: Industrial Fermentation Performance Metrics
Parameter | Value |
---|---|
Arginine Conversion | ≥85% |
Citrulline-d7 Purity | 92–95% |
By-Products | Ornithine-d5, Urea-d4 |
Downstream Processing and Purification
Post-fermentation purification involves:
-
Adsorption Decolorization : Activated carbon (1% w/v) at 60–70°C for 1 h removes pigmented impurities .
-
Vacuum Concentration : The supernatant is concentrated at 70–80°C under reduced pressure to 20–30% of its original volume .
-
Crystallization : Ethanol (3:1 v/v) is added, and the solution is cooled to 5–8°C to precipitate this compound crystals. Recrystallization from deionized water enhances purity to ≥98% .
Analytical Validation and Quality Control
Liquid Chromatography-Mass Spectrometry (LC-MS)
LC-MS with electrospray ionization (ESI) quantifies deuteration efficiency and purity. A C18 column (150 × 2.1 mm, 3.5 μm) with a gradient of 0.1% formic acid in water/acetonitrile (95:5 to 60:40 over 15 min) resolves this compound from protiated analogs . Key mass transitions:
-
This compound : m/z 182.23 [M+H]+
-
Ornithine-d5 : m/z 139.17 [M+H]+
Nuclear Magnetic Resonance (NMR) Spectroscopy
2H-NMR confirms deuterium distribution, with peaks at δ 2.95–3.10 ppm (CH2D groups) and δ 4.15 ppm (ND2) .
Properties
Molecular Formula |
C₆H₆D₇N₃O₃ |
---|---|
Molecular Weight |
182.23 |
Synonyms |
L-Citrulline-2,3,3,4,4,5,5-d7; N5-(Aminocarbonyl)-L-ornithine-d7; Citrulline-d7; N5-Carbamoyl-L-ornithine-d7; NSC 27425-d7; Nδ-Carbamylornithine-d7; α-Amino-δ-ureidovaleric Acid-d7; δ-Ureidonorvaline-d7; |
Origin of Product |
United States |
Disclaimer and Information on In-Vitro Research Products
Please be aware that all articles and product information presented on BenchChem are intended solely for informational purposes. The products available for purchase on BenchChem are specifically designed for in-vitro studies, which are conducted outside of living organisms. In-vitro studies, derived from the Latin term "in glass," involve experiments performed in controlled laboratory settings using cells or tissues. It is important to note that these products are not categorized as medicines or drugs, and they have not received approval from the FDA for the prevention, treatment, or cure of any medical condition, ailment, or disease. We must emphasize that any form of bodily introduction of these products into humans or animals is strictly prohibited by law. It is essential to adhere to these guidelines to ensure compliance with legal and ethical standards in research and experimentation.