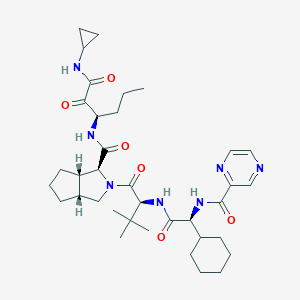
Telaprevir, (R)-
Overview
Description
Telaprevir, ®- is a direct-acting antiviral medication used in combination with other antivirals for the treatment of chronic Hepatitis C Virus infectionsSpecifically, Telaprevir inhibits the Hepatitis C viral enzyme NS3/4A serine protease, which is essential for viral replication .
Mechanism of Action
Target of Action
Telaprevir, ®- is a direct-acting antiviral medication that primarily targets the NS3/4A viral protease of the Hepatitis C Virus (HCV) . This enzyme, encoded by HCV genotype 1, is essential for viral replication .
Mode of Action
Telaprevir inhibits the NS3/4A serine protease enzyme . This inhibition prevents the cleavage of the virally encoded polyprotein into mature proteins like NS4A, NS4B, NS5A, and NS5B . This disruption in the viral life cycle prevents the virus from replicating within the host cells .
Biochemical Pathways
The primary biochemical pathway affected by Telaprevir is the replication cycle of the Hepatitis C Virus. By inhibiting the NS3/4A protease, Telaprevir prevents the maturation of viral proteins necessary for replication . This leads to a decrease in viral load and a disruption of the viral life cycle .
Pharmacokinetics
Telaprevir is well absorbed with fatty food, moderately protein-bound (59–76%), and has a large volume of distribution (~252 L) . It is primarily metabolized by cytochrome P450 (CYP) 3A4 and P-glycoprotein, and is largely excreted into feces . Telaprevir has a half-life of elimination of 4.0-4.7 hours after a single dose and an effective half-life of 9-11 hours at steady state .
Result of Action
The result of Telaprevir’s action is a significant increase in sustained virological response (SVR) in treatment-naïve patients and treatment-experienced patients . SVR and eradication of HCV infection is associated with significant long-term health benefits including reduced liver-related damage, improved quality of life, reduced incidence of Hepatocellular Carcinoma, and reduced all-cause mortality .
Action Environment
Telaprevir’s action can be influenced by environmental factors such as the presence of other medications. As a substrate and inhibitor of CYP3A4 and P-glycoprotein, Telaprevir might interact with coadministered drugs that affect or are affected by these metabolic/transport pathways . Therefore, the efficacy and stability of Telaprevir can be influenced by the patient’s medication regimen .
Biochemical Analysis
Biochemical Properties
Telaprevir, ®- plays a significant role in biochemical reactions, particularly in the inhibition of the hepatitis C viral enzyme NS3/4A serine protease . This interaction disrupts the replication cycle of the hepatitis C virus . Telaprevir is a peptidomimetic inhibitor derived based on the conformation of the viral NS5A/5B substrate, in complex with the NS3/4A protease .
Cellular Effects
Telaprevir, ®- has a profound impact on cells infected with the hepatitis C virus. It works by inhibiting the replication of the virus within the cells . This leads to a decrease in the viral load and an increase in the sustained virological response .
Molecular Mechanism
The molecular mechanism of Telaprevir, ®- involves the inhibition of the NS3/4A serine protease, an enzyme crucial for the life cycle of the hepatitis C virus . By binding to this enzyme, Telaprevir prevents the virus from replicating within the host cell .
Temporal Effects in Laboratory Settings
In laboratory settings, Telaprevir, ®- has shown to induce a median decline of more than 4 log units from baseline plasma HCV RNA levels in patients with chronic HCV genotype 1 infection over a period of 14 days .
Dosage Effects in Animal Models
In animal models, Telaprevir, ®- has shown to reduce paralysis outcomes when administered at a daily dosage of 100 mg/kg, alongside a proven dosage of 35 mg/kg . Toxicity limited doses beyond 35 mg/kg .
Metabolic Pathways
Telaprevir, ®- is primarily metabolized by cytochrome P450 (CYP) 3A4 and P-glycoprotein . It is well absorbed with fatty food, moderately protein bound (59–76 %) with a large volume of distribution (~252 L), and is largely excreted into feces .
Transport and Distribution
Telaprevir, ®- is well absorbed with fatty food, which enhances its oral bioavailability . It is primarily metabolized by cytochrome P450 (CYP) 3A4 and P-glycoprotein, and is largely excreted into feces .
Subcellular Localization
The subcellular localization of Telaprevir, ®- is currently not well established. Given its role as a protease inhibitor, it is likely to be found in the vicinity of the hepatitis C viral enzyme NS3/4A serine protease, where it exerts its inhibitory effects .
Preparation Methods
Synthetic Routes and Reaction Conditions: Telaprevir is synthesized through a series of chemical reactions involving the formation of peptide bonds and the incorporation of various functional groups. The synthesis typically involves the use of protecting groups to ensure the selective formation of the desired product. The reaction conditions often include the use of organic solvents, catalysts, and controlled temperatures to achieve high yields and purity .
Industrial Production Methods: Industrial production of Telaprevir involves large-scale synthesis using optimized reaction conditions to maximize yield and minimize production costs. The process includes the use of biocatalysis and multicomponent reactions to streamline the synthesis and improve efficiency .
Chemical Reactions Analysis
Types of Reactions: Telaprevir undergoes various chemical reactions, including oxidation, reduction, and substitution reactions. These reactions are essential for the modification of the compound’s structure to enhance its antiviral activity .
Common Reagents and Conditions: Common reagents used in the synthesis and modification of Telaprevir include organic solvents, catalysts, and protecting groups. The reaction conditions often involve controlled temperatures and pH levels to ensure the desired chemical transformations .
Major Products Formed: The major products formed from the chemical reactions involving Telaprevir include various intermediates and the final active pharmaceutical ingredient. These products are characterized by their high purity and specific structural features that contribute to the compound’s antiviral activity .
Scientific Research Applications
Telaprevir has a wide range of scientific research applications, particularly in the fields of chemistry, biology, medicine, and industry. In chemistry, it is used as a model compound for studying protease inhibitors and their mechanisms of action. In biology, Telaprevir is used to investigate the replication mechanisms of Hepatitis C Virus and the role of protease enzymes in viral infections. In medicine, Telaprevir is used as part of combination therapy for the treatment of chronic Hepatitis C Virus infections, leading to significant improvements in patient outcomes. In industry, Telaprevir serves as a benchmark for the development of new antiviral drugs and the optimization of synthetic routes for pharmaceutical production .
Comparison with Similar Compounds
- Boceprevir
- Simeprevir
- Grazoprevir
Comparison: Telaprevir, Boceprevir, Simeprevir, and Grazoprevir are all protease inhibitors used in the treatment of Hepatitis C Virus infections. Telaprevir is unique in its specific inhibition of the NS3/4A serine protease and its use in combination therapy with Ribavirin and Peginterferon alfa-2a or Peginterferon alfa-2b. This combination therapy has been shown to achieve higher sustained virological response rates compared to other protease inhibitors .
Properties
IUPAC Name |
(3S,3aS,6aR)-2-[(2S)-2-[[(2S)-2-cyclohexyl-2-(pyrazine-2-carbonylamino)acetyl]amino]-3,3-dimethylbutanoyl]-N-[(3R)-1-(cyclopropylamino)-1,2-dioxohexan-3-yl]-3,3a,4,5,6,6a-hexahydro-1H-cyclopenta[c]pyrrole-3-carboxamide | |
---|---|---|
Source | PubChem | |
URL | https://pubchem.ncbi.nlm.nih.gov | |
Description | Data deposited in or computed by PubChem | |
InChI |
InChI=1S/C36H53N7O6/c1-5-10-25(29(44)34(48)39-23-15-16-23)40-33(47)28-24-14-9-13-22(24)20-43(28)35(49)30(36(2,3)4)42-32(46)27(21-11-7-6-8-12-21)41-31(45)26-19-37-17-18-38-26/h17-19,21-25,27-28,30H,5-16,20H2,1-4H3,(H,39,48)(H,40,47)(H,41,45)(H,42,46)/t22-,24-,25+,27-,28-,30+/m0/s1 | |
Source | PubChem | |
URL | https://pubchem.ncbi.nlm.nih.gov | |
Description | Data deposited in or computed by PubChem | |
InChI Key |
BBAWEDCPNXPBQM-USXSPERKSA-N | |
Source | PubChem | |
URL | https://pubchem.ncbi.nlm.nih.gov | |
Description | Data deposited in or computed by PubChem | |
Canonical SMILES |
CCCC(C(=O)C(=O)NC1CC1)NC(=O)C2C3CCCC3CN2C(=O)C(C(C)(C)C)NC(=O)C(C4CCCCC4)NC(=O)C5=NC=CN=C5 | |
Source | PubChem | |
URL | https://pubchem.ncbi.nlm.nih.gov | |
Description | Data deposited in or computed by PubChem | |
Isomeric SMILES |
CCC[C@H](C(=O)C(=O)NC1CC1)NC(=O)[C@@H]2[C@H]3CCC[C@H]3CN2C(=O)[C@H](C(C)(C)C)NC(=O)[C@H](C4CCCCC4)NC(=O)C5=NC=CN=C5 | |
Source | PubChem | |
URL | https://pubchem.ncbi.nlm.nih.gov | |
Description | Data deposited in or computed by PubChem | |
Molecular Formula |
C36H53N7O6 | |
Source | PubChem | |
URL | https://pubchem.ncbi.nlm.nih.gov | |
Description | Data deposited in or computed by PubChem | |
Molecular Weight |
679.8 g/mol | |
Source | PubChem | |
URL | https://pubchem.ncbi.nlm.nih.gov | |
Description | Data deposited in or computed by PubChem | |
CAS No. |
923270-50-2 | |
Record name | R-Telaprevir | |
Source | ChemIDplus | |
URL | https://pubchem.ncbi.nlm.nih.gov/substance/?source=chemidplus&sourceid=0923270502 | |
Description | ChemIDplus is a free, web search system that provides access to the structure and nomenclature authority files used for the identification of chemical substances cited in National Library of Medicine (NLM) databases, including the TOXNET system. | |
Record name | VRT-127394 | |
Source | FDA Global Substance Registration System (GSRS) | |
URL | https://gsrs.ncats.nih.gov/ginas/app/beta/substances/52YTW6K39W | |
Description | The FDA Global Substance Registration System (GSRS) enables the efficient and accurate exchange of information on what substances are in regulated products. Instead of relying on names, which vary across regulatory domains, countries, and regions, the GSRS knowledge base makes it possible for substances to be defined by standardized, scientific descriptions. | |
Explanation | Unless otherwise noted, the contents of the FDA website (www.fda.gov), both text and graphics, are not copyrighted. They are in the public domain and may be republished, reprinted and otherwise used freely by anyone without the need to obtain permission from FDA. Credit to the U.S. Food and Drug Administration as the source is appreciated but not required. | |
Retrosynthesis Analysis
AI-Powered Synthesis Planning: Our tool employs the Template_relevance Pistachio, Template_relevance Bkms_metabolic, Template_relevance Pistachio_ringbreaker, Template_relevance Reaxys, Template_relevance Reaxys_biocatalysis model, leveraging a vast database of chemical reactions to predict feasible synthetic routes.
One-Step Synthesis Focus: Specifically designed for one-step synthesis, it provides concise and direct routes for your target compounds, streamlining the synthesis process.
Accurate Predictions: Utilizing the extensive PISTACHIO, BKMS_METABOLIC, PISTACHIO_RINGBREAKER, REAXYS, REAXYS_BIOCATALYSIS database, our tool offers high-accuracy predictions, reflecting the latest in chemical research and data.
Strategy Settings
Precursor scoring | Relevance Heuristic |
---|---|
Min. plausibility | 0.01 |
Model | Template_relevance |
Template Set | Pistachio/Bkms_metabolic/Pistachio_ringbreaker/Reaxys/Reaxys_biocatalysis |
Top-N result to add to graph | 6 |
Feasible Synthetic Routes
Disclaimer and Information on In-Vitro Research Products
Please be aware that all articles and product information presented on BenchChem are intended solely for informational purposes. The products available for purchase on BenchChem are specifically designed for in-vitro studies, which are conducted outside of living organisms. In-vitro studies, derived from the Latin term "in glass," involve experiments performed in controlled laboratory settings using cells or tissues. It is important to note that these products are not categorized as medicines or drugs, and they have not received approval from the FDA for the prevention, treatment, or cure of any medical condition, ailment, or disease. We must emphasize that any form of bodily introduction of these products into humans or animals is strictly prohibited by law. It is essential to adhere to these guidelines to ensure compliance with legal and ethical standards in research and experimentation.