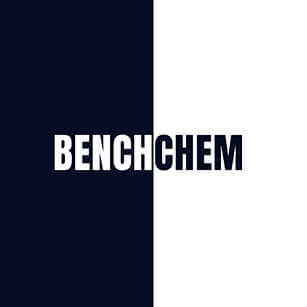
2,3-dinor Thromboxane B2-d9
- Click on QUICK INQUIRY to receive a quote from our team of experts.
- With the quality product at a COMPETITIVE price, you can focus more on your research.
Overview
Description
2,3-dinor Thromboxane B2-d9 (2,3-dinor TXB2-d9) contains 9 deuterium atoms at the 15, 15, 16, 16, 17, 17, 18, 18, and 18 positions. It is intended for use as an internal standard for the quantification of 2,3-dinor TXB2 by GC- or LC-mass spectrometry. TXB2 is released in substantial quantities from aggregating platelets and metabolized during circulation to 11-dehydro TXB2 and 2,3-dinor TXB2. 2,3-dinor TXB2 is an abundant urinary metabolite of TXB2 and can be used as a marker for in vivo TXA2 synthesis. In healthy male volunteers, the median excretion of 2,3-dinor TXB2 is 10.3 ng/hour (138 pg/mg creatine).
Scientific Research Applications
1. Method Development for Quantification
2,3-dinor Thromboxane B2-d9 has been crucial in developing methods for its quantification in human urine, specifically using gas chromatography-mass spectrometry. This method, with high accuracy, has been used to analyze urine from healthy individuals, showing relatively small diurnal and day-to-day variations in its excretion (Vesterqvist et al., 1984).
2. Understanding Urinary Metabolism
Research has shown that 2,3-dinor Thromboxane B2 is the most abundant urinary metabolite of thromboxane B2 in humans. Its measurement can help avoid acute fluctuations seen in direct measurements of thromboxane B2, thus offering a more stable indicator for monitoring thromboxane B2 release (Wilson, 1995).
3. Investigating Pathophysiological Processes
Studies have utilized 2,3-dinor Thromboxane B2 to investigate its role in various pathophysiological processes in vivo. For instance, research has noted increased urinary excretion of this metabolite in normal pregnancies and in diseases such as diabetes mellitus and homocysteinuria, compared to healthy subjects. This suggests its potential as a biomarker for these conditions (Vesterqvist & Gréen, 1984).
4. Role in Disease Contexts
In the context of diseases, 2,3-dinor Thromboxane B2 has been studied for its involvement in asthma and cardiovascular diseases. For example, research in acute asthma patients showed marked increases in urinary excretion of thromboxane-derived products, including 2,3-dinor Thromboxane B2, which were not observed in atopic volunteers after bronchial antigen challenge (Taylor et al., 1991).
5. Application in Smoking and Cardiovascular Research
The compound has been used in studies related to cardiovascular health, particularly in relation to smoking. For instance, increased excretion of 2,3-dinor Thromboxane B2 in smokers, which was reduced by aspirin administration, indicated excessive thromboxane A2 generation predominantly from platelets in smokers (Nowak et al., 1987).
Properties
Molecular Formula |
C18H21D9O6 |
---|---|
Molecular Weight |
351.5 |
InChI |
InChI=1S/C18H30O6/c1-2-3-4-7-13(19)10-11-16-14(8-5-6-9-17(21)22)15(20)12-18(23)24-16/h5-6,10-11,13-16,18-20,23H,2-4,7-9,12H2,1H3,(H,21,22)/b6-5-,11-10+/t13-,14-,15-,16+,18?/m0/s1/i1D3,2D2,3D2,4D2 |
InChI Key |
RJHNVFKNIJQTQF-NADUYOJDSA-N |
SMILES |
O[C@@H]1[C@H](C/C=CCC(O)=O)[C@@H](/C=C/[C@@H](O)CC([2H])([2H])C([2H])([2H])C([2H])([2H])C([2H])([2H])[2H])OC(O)C1 |
Synonyms |
2,3-dinor TXB2-d9 |
Origin of Product |
United States |
Retrosynthesis Analysis
AI-Powered Synthesis Planning: Our tool employs the Template_relevance Pistachio, Template_relevance Bkms_metabolic, Template_relevance Pistachio_ringbreaker, Template_relevance Reaxys, Template_relevance Reaxys_biocatalysis model, leveraging a vast database of chemical reactions to predict feasible synthetic routes.
One-Step Synthesis Focus: Specifically designed for one-step synthesis, it provides concise and direct routes for your target compounds, streamlining the synthesis process.
Accurate Predictions: Utilizing the extensive PISTACHIO, BKMS_METABOLIC, PISTACHIO_RINGBREAKER, REAXYS, REAXYS_BIOCATALYSIS database, our tool offers high-accuracy predictions, reflecting the latest in chemical research and data.
Strategy Settings
Precursor scoring | Relevance Heuristic |
---|---|
Min. plausibility | 0.01 |
Model | Template_relevance |
Template Set | Pistachio/Bkms_metabolic/Pistachio_ringbreaker/Reaxys/Reaxys_biocatalysis |
Top-N result to add to graph | 6 |
Feasible Synthetic Routes
Disclaimer and Information on In-Vitro Research Products
Please be aware that all articles and product information presented on BenchChem are intended solely for informational purposes. The products available for purchase on BenchChem are specifically designed for in-vitro studies, which are conducted outside of living organisms. In-vitro studies, derived from the Latin term "in glass," involve experiments performed in controlled laboratory settings using cells or tissues. It is important to note that these products are not categorized as medicines or drugs, and they have not received approval from the FDA for the prevention, treatment, or cure of any medical condition, ailment, or disease. We must emphasize that any form of bodily introduction of these products into humans or animals is strictly prohibited by law. It is essential to adhere to these guidelines to ensure compliance with legal and ethical standards in research and experimentation.