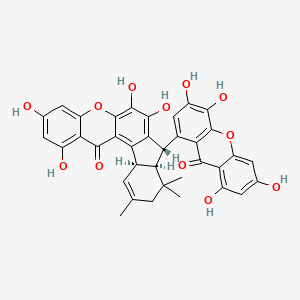
Griffipavixanthone
- Click on QUICK INQUIRY to receive a quote from our team of experts.
- With the quality product at a COMPETITIVE price, you can focus more on your research.
Overview
Description
Griffipavixanthone (GPX) is a dimeric xanthone isolated from Garcinia oblongifolia Champ . It has been found to inhibit the growth of human Non-small-cell lung cancer H520 cells in a dose- and time-dependent manner .
Synthesis Analysis
The synthesis of Griffipavixanthone has been reported in various studies . The synthesis of the natural product is accomplished via dimerization of a p-quinone methide using a chiral phosphoric acid catalyst .Molecular Structure Analysis
Griffipavixanthone has a complex molecular structure. Its chemical name is (8S,8aS,12aR)-1,3,6,7-tetrahydroxy-9,9,11-trimethyl-8-(3,4,6,8-tetrahydroxy-9-oxoxanthen-1-yl)-8,8a,10,12a-tetrahydroindeno[1,2-a]xanthen-13-one .Chemical Reactions Analysis
The synthesis of xanthones like Griffipavixanthone involves various chemical reactions. These include the classical and the modified Grover, Shah, and Shah reaction; the use of ytterbium, palladium, ruthenium, copper catalysis; the use of chromen-4-ones as building blocks; the use of the Friedel–Crafts reaction; Ullmann-ether coupling; metal-free oxidative coupling; intermolecular and intramolecular couplings .Physical And Chemical Properties Analysis
Griffipavixanthone is a yellow powder with a molecular weight of 652.6. It is soluble in Chloroform, Dichloromethane, Ethyl Acetate, DMSO, Acetone, etc .Scientific Research Applications
Anti-Cancer Activity
GPX has been found to have significant anti-cancer properties. It has been shown to inhibit the growth of H520 cells, a type of human non-small-cell lung cancer (NSCLC), in a dose- and time-dependent manner . The IC50 values, which indicate the concentration of GPX required to inhibit cell growth by 50%, were found to be 3.03 ± 0.21 μM at 48 hours .
Induction of Apoptosis
GPX induces apoptosis, or programmed cell death, in cancer cells. This was observed in H520 cells treated with GPX . The morphologic characteristics of apoptosis and apoptotic bodies were observed under a fluorescence microscope and transmission electron microscope .
Effect on Mitochondrial Membrane Potential
GPX has been found to reduce the mitochondrial membrane potential . This is significant because the mitochondrial membrane potential is critical for maintaining the function of the mitochondria, the powerhouse of the cell. Disruption of this potential can lead to cell death.
Generation of Reactive Oxygen Species (ROS)
GPX treatment has been shown to increase the production of intracellular ROS . ROS are chemically reactive molecules that contain oxygen. While they play important roles in cell signaling and homeostasis, excessive amounts can lead to significant damage to cell structures, a phenomenon known as oxidative stress.
Activation of Caspase-3
GPX has been found to cleave and activate caspase-3 . Caspase-3 is a critical executioner of apoptosis, as it is responsible for the proteolytic cleavage of many key proteins.
Suppression of Pulmonary Metastasis
In vivo studies have indicated that GPX can suppress pulmonary metastasis without significant side effects against other organs in nude mice . This suggests that GPX could potentially be used as a therapeutic agent in the treatment of lung cancer metastasis.
Mechanism of Action
Target of Action
Griffipavixanthone (GPX), a dimeric xanthone isolated from Garcinia esculenta, primarily targets the RAF proteins . The RAF family proteins play a crucial role in promoting tumor metastasis and proliferation . GPX has been identified as a B-RAF and C-RAF inhibitor against esophageal cancer cells .
Mode of Action
GPX interacts with its targets, the RAF proteins, leading to their inhibition . This inhibition significantly reduces cell migration and invasion, as confirmed by wound healing, transwell migration, and matrigel invasion assays . Furthermore, exposure to GPX induces G2/M cell cycle arrest .
Biochemical Pathways
The primary biochemical pathway affected by GPX is the RAF-MEK-ERK cascade . GPX suppresses cancer metastasis and proliferation through downregulation of RAF-MEK-ERK cascade proteins as well as RAF mRNA levels . This pathway is involved in cell proliferation, metastasis, and cell survival .
Pharmacokinetics
The bioavailability of gpx is suggested by its significant effects on cell proliferation and apoptosis in vitro .
Result of Action
The action of GPX results in significant molecular and cellular effects. It inhibits cell proliferation in a dose- and time-dependent manner . GPX also induces cell apoptosis through the mitochondrial apoptotic pathway, accompanied by reactive oxygen species (ROS) production . In addition, GPX cleaves and activates caspase-3 .
Action Environment
The significant effects of gpx on cell proliferation and apoptosis in vitro suggest that it may be effective in a variety of cellular environments .
Safety and Hazards
properties
IUPAC Name |
(8S,8aS,12aR)-1,3,6,7-tetrahydroxy-9,9,11-trimethyl-8-(3,4,6,8-tetrahydroxy-9-oxoxanthen-1-yl)-8,8a,10,12a-tetrahydroindeno[1,2-a]xanthen-13-one |
Source
|
---|---|---|
Source | PubChem | |
URL | https://pubchem.ncbi.nlm.nih.gov | |
Description | Data deposited in or computed by PubChem | |
InChI |
InChI=1S/C36H28O12/c1-11-4-15-21-26(32(45)33(46)35-27(21)31(44)25-17(40)6-13(38)8-20(25)48-35)22(28(15)36(2,3)10-11)14-9-18(41)29(42)34-23(14)30(43)24-16(39)5-12(37)7-19(24)47-34/h4-9,15,22,28,37-42,45-46H,10H2,1-3H3/t15-,22-,28-/m0/s1 |
Source
|
Source | PubChem | |
URL | https://pubchem.ncbi.nlm.nih.gov | |
Description | Data deposited in or computed by PubChem | |
InChI Key |
KAPVRSRPLHVWCH-JNFGOLMQSA-N |
Source
|
Source | PubChem | |
URL | https://pubchem.ncbi.nlm.nih.gov | |
Description | Data deposited in or computed by PubChem | |
Canonical SMILES |
CC1=CC2C(C(C3=C(C(=C4C(=C23)C(=O)C5=C(C=C(C=C5O4)O)O)O)O)C6=CC(=C(C7=C6C(=O)C8=C(C=C(C=C8O7)O)O)O)O)C(C1)(C)C |
Source
|
Source | PubChem | |
URL | https://pubchem.ncbi.nlm.nih.gov | |
Description | Data deposited in or computed by PubChem | |
Isomeric SMILES |
CC1=C[C@@H]2[C@@H]([C@H](C3=C(C(=C4C(=C23)C(=O)C5=C(C=C(C=C5O4)O)O)O)O)C6=CC(=C(C7=C6C(=O)C8=C(C=C(C=C8O7)O)O)O)O)C(C1)(C)C |
Source
|
Source | PubChem | |
URL | https://pubchem.ncbi.nlm.nih.gov | |
Description | Data deposited in or computed by PubChem | |
Molecular Formula |
C36H28O12 |
Source
|
Source | PubChem | |
URL | https://pubchem.ncbi.nlm.nih.gov | |
Description | Data deposited in or computed by PubChem | |
Molecular Weight |
652.6 g/mol |
Source
|
Source | PubChem | |
URL | https://pubchem.ncbi.nlm.nih.gov | |
Description | Data deposited in or computed by PubChem | |
Disclaimer and Information on In-Vitro Research Products
Please be aware that all articles and product information presented on BenchChem are intended solely for informational purposes. The products available for purchase on BenchChem are specifically designed for in-vitro studies, which are conducted outside of living organisms. In-vitro studies, derived from the Latin term "in glass," involve experiments performed in controlled laboratory settings using cells or tissues. It is important to note that these products are not categorized as medicines or drugs, and they have not received approval from the FDA for the prevention, treatment, or cure of any medical condition, ailment, or disease. We must emphasize that any form of bodily introduction of these products into humans or animals is strictly prohibited by law. It is essential to adhere to these guidelines to ensure compliance with legal and ethical standards in research and experimentation.