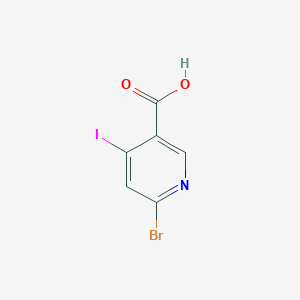
6-Bromo-4-iodonicotinic acid
Overview
Description
6-Bromo-4-iodonicotinic acid is an organic compound with the molecular formula C6H3BrINO2 It is a derivative of nicotinic acid, characterized by the presence of bromine and iodine atoms at the 6th and 4th positions, respectively
Mechanism of Action
Mode of Action
It’s possible that it interacts with its targets via covalent bonding, given the presence of the bromine and iodine atoms, which are known for their electrophilic properties. This is purely speculative and requires experimental validation .
Biochemical Pathways
The biochemical pathways affected by 6-Bromo-4-iodonicotinic acid are currently unknown . It’s worth noting that bromine and iodine-containing compounds are often used in suzuki–miyaura cross-coupling reactions, a widely-applied transition metal catalysed carbon–carbon bond forming reaction . This suggests that this compound could potentially be involved in similar biochemical pathways.
Preparation Methods
Synthetic Routes and Reaction Conditions
The synthesis of 6-Bromo-4-iodonicotinic acid typically involves halogenation reactions. One common method is the bromination of 4-iodonicotinic acid. The reaction is carried out using bromine or a brominating agent such as N-bromosuccinimide (NBS) in the presence of a catalyst or under specific reaction conditions to achieve selective bromination at the 6th position.
Industrial Production Methods
While specific industrial production methods for this compound are not widely documented, the general approach would involve scaling up the laboratory synthesis methods. This includes optimizing reaction conditions, using efficient catalysts, and ensuring high purity and yield through purification techniques such as recrystallization or chromatography.
Chemical Reactions Analysis
Types of Reactions
6-Bromo-4-iodonicotinic acid can undergo various chemical reactions, including:
Substitution Reactions: The bromine and iodine atoms can be substituted with other functional groups through nucleophilic substitution reactions.
Coupling Reactions: It can participate in Suzuki-Miyaura coupling reactions, where the halogen atoms are replaced with aryl or alkyl groups using palladium catalysts and boronic acids.
Oxidation and Reduction: The compound can undergo oxidation or reduction reactions to modify its functional groups or oxidation state.
Common Reagents and Conditions
Nucleophilic Substitution: Reagents such as sodium azide or potassium thiolate can be used under mild conditions.
Suzuki-Miyaura Coupling: Palladium catalysts, boronic acids, and bases like potassium carbonate are commonly used.
Oxidation: Oxidizing agents like potassium permanganate or chromium trioxide can be employed.
Major Products
The major products formed from these reactions depend on the specific reagents and conditions used. For example, Suzuki-Miyaura coupling can yield various substituted nicotinic acids, while nucleophilic substitution can introduce different functional groups at the halogen positions.
Scientific Research Applications
6-Bromo-4-iodonicotinic acid has several scientific research applications:
Organic Synthesis: It serves as a building block for the synthesis of more complex organic molecules, particularly in the development of pharmaceuticals and agrochemicals.
Medicinal Chemistry: The compound is used in the design and synthesis of potential drug candidates, especially those targeting specific biological pathways.
Material Science: It can be used in the synthesis of novel materials with unique properties, such as conducting polymers or coordination complexes.
Biological Studies: Researchers use it to study the effects of halogenated nicotinic acid derivatives on biological systems, including their potential as enzyme inhibitors or receptor modulators.
Comparison with Similar Compounds
Similar Compounds
4-Iodonicotinic Acid: Lacks the bromine atom at the 6th position, which may affect its reactivity and binding properties.
6-Bromo-4-chloronicotinic Acid: Contains a chlorine atom instead of iodine, which can influence its chemical behavior and applications.
6-Bromo-4-fluoronicotinic Acid: The presence of fluorine instead of iodine can lead to different electronic and steric effects.
Uniqueness
6-Bromo-4-iodonicotinic acid is unique due to the combination of bromine and iodine atoms, which can provide distinct reactivity and binding characteristics compared to other halogenated nicotinic acids. This makes it a valuable compound for specific synthetic and research applications.
Properties
IUPAC Name |
6-bromo-4-iodopyridine-3-carboxylic acid | |
---|---|---|
Source | PubChem | |
URL | https://pubchem.ncbi.nlm.nih.gov | |
Description | Data deposited in or computed by PubChem | |
InChI |
InChI=1S/C6H3BrINO2/c7-5-1-4(8)3(2-9-5)6(10)11/h1-2H,(H,10,11) | |
Source | PubChem | |
URL | https://pubchem.ncbi.nlm.nih.gov | |
Description | Data deposited in or computed by PubChem | |
InChI Key |
YOGAGPKWRKIOFY-UHFFFAOYSA-N | |
Source | PubChem | |
URL | https://pubchem.ncbi.nlm.nih.gov | |
Description | Data deposited in or computed by PubChem | |
Canonical SMILES |
C1=C(C(=CN=C1Br)C(=O)O)I | |
Source | PubChem | |
URL | https://pubchem.ncbi.nlm.nih.gov | |
Description | Data deposited in or computed by PubChem | |
Molecular Formula |
C6H3BrINO2 | |
Source | PubChem | |
URL | https://pubchem.ncbi.nlm.nih.gov | |
Description | Data deposited in or computed by PubChem | |
Molecular Weight |
327.90 g/mol | |
Source | PubChem | |
URL | https://pubchem.ncbi.nlm.nih.gov | |
Description | Data deposited in or computed by PubChem | |
Synthesis routes and methods
Procedure details
Disclaimer and Information on In-Vitro Research Products
Please be aware that all articles and product information presented on BenchChem are intended solely for informational purposes. The products available for purchase on BenchChem are specifically designed for in-vitro studies, which are conducted outside of living organisms. In-vitro studies, derived from the Latin term "in glass," involve experiments performed in controlled laboratory settings using cells or tissues. It is important to note that these products are not categorized as medicines or drugs, and they have not received approval from the FDA for the prevention, treatment, or cure of any medical condition, ailment, or disease. We must emphasize that any form of bodily introduction of these products into humans or animals is strictly prohibited by law. It is essential to adhere to these guidelines to ensure compliance with legal and ethical standards in research and experimentation.