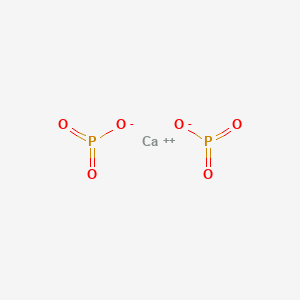
Calcium metaphosphate
Overview
Description
Calcium metaphosphate is an inorganic compound with the chemical formula ( \text{Ca(PO}_3\text{)}_2 ). It is a type of phosphate glass that is known for its unique properties, including its ability to form a glassy, amorphous structure. This compound is commonly used in various industrial and scientific applications due to its stability and bioactivity.
Preparation Methods
Synthetic Routes and Reaction Conditions: Calcium metaphosphate can be synthesized through several methods. One common method involves the reaction of calcium oxide (CaO) with phosphoric acid (H₃PO₄) at high temperatures. The reaction typically takes place in a furnace at temperatures ranging from 800°C to 1000°C. The resulting product is then cooled rapidly to form a glassy, amorphous structure.
Industrial Production Methods: In industrial settings, this compound is often produced using a melt-quenching technique. This involves melting a mixture of calcium oxide and phosphoric acid in a high-temperature furnace and then rapidly cooling the melt to form a glass. Another method involves the use of a sol-gel process, where a solution containing calcium and phosphate precursors is gelled and then heated to form the desired compound .
Chemical Reactions Analysis
Types of Reactions: Calcium metaphosphate undergoes various chemical reactions, including hydrolysis, where it reacts with water to form orthophosphate. It can also participate in ion-exchange reactions, where calcium ions are replaced by other cations.
Common Reagents and Conditions:
Hydrolysis: In the presence of water, this compound can hydrolyze to form calcium orthophosphate and phosphoric acid.
Ion-Exchange Reactions: this compound can react with solutions containing other cations, such as sodium or potassium, leading to the exchange of calcium ions with these cations.
Major Products Formed:
Hydrolysis: Calcium orthophosphate and phosphoric acid.
Ion-Exchange Reactions: Sodium metaphosphate or potassium metaphosphate, depending on the cation used .
Scientific Research Applications
Calcium metaphosphate has a wide range of applications in scientific research, particularly in the fields of chemistry, biology, medicine, and industry.
Chemistry:
- Used as a precursor for the synthesis of other phosphate compounds.
- Employed in the study of glassy and amorphous materials.
Biology and Medicine:
- Utilized in bone regeneration and repair due to its bioactive properties.
- Applied in the development of bioactive coatings for implants to enhance their integration with bone tissue .
Industry:
- Used in the production of specialty glasses and ceramics.
- Employed as a component in fertilizers due to its phosphorus content .
Mechanism of Action
The mechanism of action of calcium metaphosphate in biological systems involves the release of calcium and phosphate ions. These ions play a crucial role in various physiological processes, including bone mineralization and cellular signaling. The release of calcium ions can activate osteoblasts, promoting bone formation, while phosphate ions contribute to the mineralization process .
Comparison with Similar Compounds
Hydroxyapatite: Known for its high bioactivity and similarity to natural bone mineral. It is widely used in bone grafts and dental applications.
Tricalcium Phosphate: Used in bone grafts and as a calcium supplement. It has a higher solubility compared to hydroxyapatite.
Dicalcium Phosphate: Commonly used as a dietary supplement and in the production of animal feed.
Uniqueness of Calcium Metaphosphate:
- Forms a glassy, amorphous structure, unlike the crystalline nature of hydroxyapatite and tricalcium phosphate.
- Exhibits unique ion-exchange properties, making it useful in various industrial applications.
- Its ability to form bioactive coatings enhances its application in medical implants .
Properties
InChI |
InChI=1S/Ca.2HO3P/c;2*1-4(2)3/h;2*(H,1,2,3)/q+2;;/p-2 | |
---|---|---|
Source | PubChem | |
URL | https://pubchem.ncbi.nlm.nih.gov | |
Description | Data deposited in or computed by PubChem | |
InChI Key |
ROPDWRCJTIRLTR-UHFFFAOYSA-L | |
Source | PubChem | |
URL | https://pubchem.ncbi.nlm.nih.gov | |
Description | Data deposited in or computed by PubChem | |
Canonical SMILES |
[O-]P(=O)=O.[O-]P(=O)=O.[Ca+2] | |
Source | PubChem | |
URL | https://pubchem.ncbi.nlm.nih.gov | |
Description | Data deposited in or computed by PubChem | |
Molecular Formula |
CaO6P2 | |
Source | PubChem | |
URL | https://pubchem.ncbi.nlm.nih.gov | |
Description | Data deposited in or computed by PubChem | |
DSSTOX Substance ID |
DTXSID10872573 | |
Record name | Calcium bis(metaphosphate) | |
Source | EPA DSSTox | |
URL | https://comptox.epa.gov/dashboard/DTXSID10872573 | |
Description | DSSTox provides a high quality public chemistry resource for supporting improved predictive toxicology. | |
Molecular Weight |
198.02 g/mol | |
Source | PubChem | |
URL | https://pubchem.ncbi.nlm.nih.gov | |
Description | Data deposited in or computed by PubChem | |
Physical Description |
Odourless, colourless crystals or white powder | |
Record name | CALCIUM POLYPHOSPHATE | |
Source | EU Food Improvement Agents | |
URL | https://eur-lex.europa.eu/legal-content/EN/ALL/?uri=CELEX%3A32012R0231 | |
Description | Commission Regulation (EU) No 231/2012 of 9 March 2012 laying down specifications for food additives listed in Annexes II and III to Regulation (EC) No 1333/2008 of the European Parliament and of the Council Text with EEA relevance | |
Solubility |
Usually sparingly soluble in water. Soluble in acid medium | |
Record name | CALCIUM POLYPHOSPHATE | |
Source | EU Food Improvement Agents | |
URL | https://eur-lex.europa.eu/legal-content/EN/ALL/?uri=CELEX%3A32012R0231 | |
Description | Commission Regulation (EU) No 231/2012 of 9 March 2012 laying down specifications for food additives listed in Annexes II and III to Regulation (EC) No 1333/2008 of the European Parliament and of the Council Text with EEA relevance | |
CAS No. |
13477-39-9, 53801-86-8, 123093-85-6 | |
Record name | Metaphosphoric acid (HPO3), calcium salt (2:1) | |
Source | ChemIDplus | |
URL | https://pubchem.ncbi.nlm.nih.gov/substance/?source=chemidplus&sourceid=0013477399 | |
Description | ChemIDplus is a free, web search system that provides access to the structure and nomenclature authority files used for the identification of chemical substances cited in National Library of Medicine (NLM) databases, including the TOXNET system. | |
Record name | Metaphosphoric acid, calcium salt | |
Source | ChemIDplus | |
URL | https://pubchem.ncbi.nlm.nih.gov/substance/?source=chemidplus&sourceid=0053801868 | |
Description | ChemIDplus is a free, web search system that provides access to the structure and nomenclature authority files used for the identification of chemical substances cited in National Library of Medicine (NLM) databases, including the TOXNET system. | |
Record name | Calcium metaphosphate | |
Source | ChemIDplus | |
URL | https://pubchem.ncbi.nlm.nih.gov/substance/?source=chemidplus&sourceid=0123093856 | |
Description | ChemIDplus is a free, web search system that provides access to the structure and nomenclature authority files used for the identification of chemical substances cited in National Library of Medicine (NLM) databases, including the TOXNET system. | |
Record name | Metaphosphoric acid (HPO3), calcium salt (2:1) | |
Source | EPA Chemicals under the TSCA | |
URL | https://www.epa.gov/chemicals-under-tsca | |
Description | EPA Chemicals under the Toxic Substances Control Act (TSCA) collection contains information on chemicals and their regulations under TSCA, including non-confidential content from the TSCA Chemical Substance Inventory and Chemical Data Reporting. | |
Record name | Calcium bis(metaphosphate) | |
Source | EPA DSSTox | |
URL | https://comptox.epa.gov/dashboard/DTXSID10872573 | |
Description | DSSTox provides a high quality public chemistry resource for supporting improved predictive toxicology. | |
Record name | Calcium bis(metaphosphate) | |
Source | European Chemicals Agency (ECHA) | |
URL | https://echa.europa.eu/substance-information/-/substanceinfo/100.033.412 | |
Description | The European Chemicals Agency (ECHA) is an agency of the European Union which is the driving force among regulatory authorities in implementing the EU's groundbreaking chemicals legislation for the benefit of human health and the environment as well as for innovation and competitiveness. | |
Explanation | Use of the information, documents and data from the ECHA website is subject to the terms and conditions of this Legal Notice, and subject to other binding limitations provided for under applicable law, the information, documents and data made available on the ECHA website may be reproduced, distributed and/or used, totally or in part, for non-commercial purposes provided that ECHA is acknowledged as the source: "Source: European Chemicals Agency, http://echa.europa.eu/". Such acknowledgement must be included in each copy of the material. ECHA permits and encourages organisations and individuals to create links to the ECHA website under the following cumulative conditions: Links can only be made to webpages that provide a link to the Legal Notice page. | |
Synthesis routes and methods I
Procedure details
Synthesis routes and methods II
Procedure details
Disclaimer and Information on In-Vitro Research Products
Please be aware that all articles and product information presented on BenchChem are intended solely for informational purposes. The products available for purchase on BenchChem are specifically designed for in-vitro studies, which are conducted outside of living organisms. In-vitro studies, derived from the Latin term "in glass," involve experiments performed in controlled laboratory settings using cells or tissues. It is important to note that these products are not categorized as medicines or drugs, and they have not received approval from the FDA for the prevention, treatment, or cure of any medical condition, ailment, or disease. We must emphasize that any form of bodily introduction of these products into humans or animals is strictly prohibited by law. It is essential to adhere to these guidelines to ensure compliance with legal and ethical standards in research and experimentation.