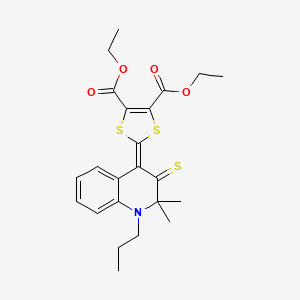
diethyl 2-(2,2-dimethyl-1-propyl-3-thioxo-2,3-dihydroquinolin-4(1H)-ylidene)-1,3-dithiole-4,5-dicarboxylate
- Click on QUICK INQUIRY to receive a quote from our team of experts.
- With the quality product at a COMPETITIVE price, you can focus more on your research.
Overview
Description
Diethyl 2-(2,2-dimethyl-1-propyl-3-thioxo-2,3-dihydroquinolin-4(1H)-ylidene)-1,3-dithiole-4,5-dicarboxylate is a complex organic compound that features a quinoline core with various functional groups attached
Preparation Methods
Synthetic Routes and Reaction Conditions
The synthesis of diethyl 2-(2,2-dimethyl-1-propyl-3-thioxo-2,3-dihydroquinolin-4(1H)-ylidene)-1,3-dithiole-4,5-dicarboxylate typically involves multiple steps:
Formation of the Quinoline Core: The quinoline core can be synthesized through a Friedländer synthesis, which involves the condensation of aniline derivatives with carbonyl compounds under acidic or basic conditions.
Introduction of the Thioxo Group: The thioxo group can be introduced via a thiolation reaction, where a suitable thiol reagent is used.
Formation of the Dithiole Ring: The dithiole ring can be formed through a cyclization reaction involving dithiocarboxylate esters.
Final Coupling: The final step involves coupling the quinoline and dithiole moieties under specific conditions to yield the target compound.
Industrial Production Methods
Industrial production of this compound would likely involve optimization of the above synthetic routes to ensure high yield and purity. This may include the use of catalysts, controlled reaction environments, and purification techniques such as recrystallization or chromatography.
Chemical Reactions Analysis
Types of Reactions
Oxidation: The compound can undergo oxidation reactions, particularly at the thioxo group, leading to the formation of sulfoxides or sulfones.
Reduction: Reduction reactions can target the quinoline core or the thioxo group, potentially yielding dihydroquinoline derivatives or thiol groups.
Substitution: The compound can participate in substitution reactions, where functional groups on the quinoline or dithiole rings are replaced by other groups.
Common Reagents and Conditions
Oxidation: Common oxidizing agents include hydrogen peroxide, m-chloroperbenzoic acid, and potassium permanganate.
Reduction: Reducing agents such as sodium borohydride, lithium aluminum hydride, or catalytic hydrogenation can be used.
Substitution: Reagents like halogens, alkylating agents, or nucleophiles can facilitate substitution reactions.
Major Products
Oxidation: Sulfoxides, sulfones
Reduction: Dihydroquinoline derivatives, thiol groups
Substitution: Various substituted quinoline and dithiole derivatives
Scientific Research Applications
Chemistry
Catalysis: The compound can be used as a ligand in coordination chemistry, potentially serving as a catalyst in various organic reactions.
Materials Science: Its unique structure may allow it to be used in the development of novel materials with specific electronic or optical properties.
Biology and Medicine
Drug Development: The compound’s quinoline core makes it a candidate for drug development, particularly in the treatment of diseases such as malaria or cancer.
Biological Probes: It can be used as a probe in biological studies to investigate enzyme activities or cellular processes.
Industry
Dyes and Pigments: The compound’s structure may allow it to be used in the synthesis of dyes and pigments with specific color properties.
Polymer Additives: It can be used as an additive in polymers to enhance their properties, such as stability or conductivity.
Mechanism of Action
The mechanism by which diethyl 2-(2,2-dimethyl-1-propyl-3-thioxo-2,3-dihydroquinolin-4(1H)-ylidene)-1,3-dithiole-4,5-dicarboxylate exerts its effects depends on its application:
Catalysis: As a ligand, it can coordinate with metal centers, facilitating various catalytic processes.
Drug Action: In medicinal applications, it may interact with specific molecular targets such as enzymes or receptors, modulating their activity and leading to therapeutic effects.
Comparison with Similar Compounds
Similar Compounds
Quinoline Derivatives: Compounds such as chloroquine and quinine share the quinoline core and are used in medicinal chemistry.
Dithiole Derivatives: Compounds like dithiolethiones are known for their antioxidant properties and potential therapeutic applications.
Uniqueness
Diethyl 2-(2,2-dimethyl-1-propyl-3-thioxo-2,3-dihydroquinolin-4(1H)-ylidene)-1,3-dithiole-4,5-dicarboxylate is unique due to the combination of the quinoline and dithiole moieties, which imparts distinct chemical and biological properties not found in simpler analogs.
Biological Activity
Diethyl 2-(2,2-dimethyl-1-propyl-3-thioxo-2,3-dihydroquinolin-4(1H)-ylidene)-1,3-dithiole-4,5-dicarboxylate is a complex organic compound with significant biological activity. This article provides a comprehensive overview of its biological properties, including its synthesis, mechanisms of action, and potential therapeutic applications.
Chemical Structure and Properties
The compound's molecular formula is C21H23NO4S3, with a molecular weight of approximately 449.6 g/mol. Its structure includes a dithiole ring, which is known for its pharmacological properties due to the presence of sulfur atoms that can interact with biological systems.
Property | Value |
---|---|
Molecular Formula | C21H23NO4S3 |
Molecular Weight | 449.6 g/mol |
InChI Key | SYYWPNCFTAGNOA-UHFFFAOYSA-N |
Exact Mass | 449.078922 g/mol |
Anticancer Properties
Research indicates that compounds containing dithiolethione structures exhibit anticancer activity. A study highlighted that dithiolethiones can induce apoptosis in cancer cells through the activation of mitochondrial pathways and the inhibition of cell proliferation. The compound's ability to modulate various signaling pathways contributes to its potential as an anticancer agent .
Antioxidant Activity
Dithiolethiones are recognized for their antioxidative properties , which help in scavenging free radicals and reducing oxidative stress in cells. This activity is crucial in preventing cellular damage associated with various diseases, including cancer and neurodegenerative disorders .
Anti-inflammatory Effects
Preliminary studies suggest that the compound may exhibit anti-inflammatory effects by inhibiting the production of pro-inflammatory cytokines and mediators such as nitric oxide (NO). The inhibition of inducible nitric oxide synthase (iNOS) has been linked to these anti-inflammatory properties, making it a candidate for further investigation in inflammatory diseases .
The biological activities of this compound are primarily attributed to:
- Interaction with Cellular Targets : The compound may interact with various cellular targets involved in signaling pathways that regulate cell growth and apoptosis.
- Modulation of Enzymatic Activity : It has been shown to inhibit certain enzymes related to inflammation and cancer progression.
- Induction of Oxidative Stress : By modulating redox status within cells, it can promote apoptosis in cancer cells while protecting normal cells from oxidative damage.
Case Studies
- In Vitro Studies : A series of in vitro experiments demonstrated that derivatives of dithiolethiones exhibit significant cytotoxicity against various cancer cell lines. The mechanism was linked to the induction of oxidative stress leading to apoptosis .
- Animal Models : In vivo studies have indicated that compounds similar to this compound can reduce tumor size in animal models when administered at specific dosages .
Properties
Molecular Formula |
C23H27NO4S3 |
---|---|
Molecular Weight |
477.7 g/mol |
IUPAC Name |
diethyl 2-(2,2-dimethyl-1-propyl-3-sulfanylidenequinolin-4-ylidene)-1,3-dithiole-4,5-dicarboxylate |
InChI |
InChI=1S/C23H27NO4S3/c1-6-13-24-15-12-10-9-11-14(15)16(19(29)23(24,4)5)22-30-17(20(25)27-7-2)18(31-22)21(26)28-8-3/h9-12H,6-8,13H2,1-5H3 |
InChI Key |
KCUACFMIWQXYLF-UHFFFAOYSA-N |
Canonical SMILES |
CCCN1C2=CC=CC=C2C(=C3SC(=C(S3)C(=O)OCC)C(=O)OCC)C(=S)C1(C)C |
Origin of Product |
United States |
Disclaimer and Information on In-Vitro Research Products
Please be aware that all articles and product information presented on BenchChem are intended solely for informational purposes. The products available for purchase on BenchChem are specifically designed for in-vitro studies, which are conducted outside of living organisms. In-vitro studies, derived from the Latin term "in glass," involve experiments performed in controlled laboratory settings using cells or tissues. It is important to note that these products are not categorized as medicines or drugs, and they have not received approval from the FDA for the prevention, treatment, or cure of any medical condition, ailment, or disease. We must emphasize that any form of bodily introduction of these products into humans or animals is strictly prohibited by law. It is essential to adhere to these guidelines to ensure compliance with legal and ethical standards in research and experimentation.