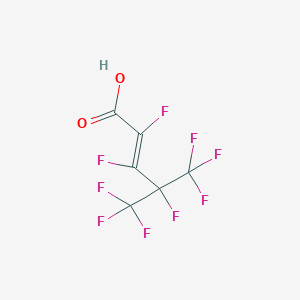
PERFLUORO(4-METHYLPENT-2-ENOIC ACID)
Overview
Description
4-(Trifluoromethyl)hexafluoropent-2-enoic acid is a fluorinated organic compound with the molecular formula C6HF9O2. It is characterized by the presence of both trifluoromethyl and hexafluoro groups, making it a highly fluorinated molecule. This compound is known for its unique chemical properties, which are influenced by the presence of multiple fluorine atoms.
Mechanism of Action
Mode of Action
It has been observed that the compound undergoes electrochemical reduction . This process occurs at an applied cell potential of 10 V in the absence of an electron shuttling catalyst .
Biochemical Pathways
It is known that the compound undergoes electrochemical reduction , which could potentially impact various biochemical processes.
Result of Action
It has been observed that the compound undergoes electrochemical reduction , which could potentially lead to various molecular and cellular changes.
Biochemical Analysis
Biochemical Properties
Perfluoro(4-methylpent-2-enoic acid): plays a significant role in biochemical reactions, particularly in the context of its interactions with enzymes and proteins. It has been observed to interact with enzymes involved in defluorination processes, such as reductive defluorinases . These enzymes facilitate the removal of fluorine atoms from the compound, leading to its breakdown. The interaction between Perfluoro(4-methylpent-2-enoic acid) and these enzymes is crucial for understanding its metabolic fate and potential environmental impact .
Cellular Effects
The effects of Perfluoro(4-methylpent-2-enoic acid) on various cell types and cellular processes have been studied extensively. This compound has been shown to influence cell signaling pathways, particularly those involved in oxidative stress responses. It can alter gene expression patterns, leading to changes in cellular metabolism and function. For instance, exposure to Perfluoro(4-methylpent-2-enoic acid) can result in the upregulation of genes associated with detoxification processes, highlighting its impact on cellular defense mechanisms .
Molecular Mechanism
At the molecular level, Perfluoro(4-methylpent-2-enoic acid) exerts its effects through specific binding interactions with biomolecules. It can inhibit certain enzymes by binding to their active sites, thereby preventing their normal function. Additionally, this compound can activate other enzymes, leading to enhanced metabolic activity. The changes in gene expression observed with Perfluoro(4-methylpent-2-enoic acid) exposure are often mediated by transcription factors that respond to oxidative stress and other cellular signals .
Temporal Effects in Laboratory Settings
In laboratory settings, the effects of Perfluoro(4-methylpent-2-enoic acid) have been observed to change over time. The compound is relatively stable, but it can undergo degradation under certain conditions, such as exposure to high temperatures or specific microbial communities. Long-term studies have shown that Perfluoro(4-methylpent-2-enoic acid) can have persistent effects on cellular function, including sustained changes in gene expression and metabolic activity .
Dosage Effects in Animal Models
The effects of Perfluoro(4-methylpent-2-enoic acid) vary with different dosages in animal models. At low doses, the compound may have minimal impact on cellular function, while higher doses can lead to significant toxic effects. These effects include oxidative stress, inflammation, and disruption of normal metabolic processes. Threshold effects have been observed, where a certain dosage level must be reached before adverse effects become apparent .
Metabolic Pathways
Perfluoro(4-methylpent-2-enoic acid): is involved in several metabolic pathways, primarily those related to its defluorination and breakdown. Enzymes such as reductive defluorinases play a key role in these pathways, facilitating the removal of fluorine atoms and the subsequent degradation of the compound. The interaction of Perfluoro(4-methylpent-2-enoic acid) with these enzymes can affect metabolic flux and the levels of various metabolites .
Transport and Distribution
Within cells and tissues, Perfluoro(4-methylpent-2-enoic acid) is transported and distributed through interactions with specific transporters and binding proteins. These interactions can influence the localization and accumulation of the compound, affecting its overall bioavailability and activity. The transport and distribution of Perfluoro(4-methylpent-2-enoic acid) are critical for understanding its pharmacokinetics and potential therapeutic applications .
Subcellular Localization
The subcellular localization of Perfluoro(4-methylpent-2-enoic acid) is influenced by targeting signals and post-translational modifications. These factors direct the compound to specific compartments or organelles within the cell, where it can exert its effects. For example, Perfluoro(4-methylpent-2-enoic acid) may accumulate in the mitochondria, leading to changes in mitochondrial function and energy metabolism .
Preparation Methods
Synthetic Routes and Reaction Conditions: The synthesis of 4-(Trifluoromethyl)hexafluoropent-2-enoic acid typically involves the introduction of fluorine atoms into the molecular structure through various fluorination reactions. One common method involves the reaction of a suitable precursor with fluorinating agents such as sulfur tetrafluoride or cobalt trifluoride under controlled conditions. The reaction is usually carried out in an inert atmosphere to prevent unwanted side reactions.
Industrial Production Methods: Industrial production of this compound may involve large-scale fluorination processes using specialized equipment to handle the highly reactive fluorinating agents. The reaction conditions, including temperature, pressure, and reaction time, are optimized to achieve high yields and purity of the final product.
Chemical Reactions Analysis
Types of Reactions: 4-(Trifluoromethyl)hexafluoropent-2-enoic acid can undergo various types of chemical reactions, including:
Oxidation: The compound can be oxidized to form corresponding perfluorinated carboxylic acids.
Reduction: Reduction reactions can lead to the formation of partially fluorinated derivatives.
Substitution: The fluorine atoms in the compound can be substituted with other functional groups under specific conditions.
Common Reagents and Conditions:
Oxidation: Common oxidizing agents include potassium permanganate and hydrogen peroxide.
Reduction: Reducing agents such as lithium aluminum hydride or sodium borohydride are used.
Substitution: Nucleophilic substitution reactions can be carried out using reagents like sodium methoxide or potassium tert-butoxide.
Major Products Formed: The major products formed from these reactions depend on the specific reagents and conditions used. For example, oxidation may yield perfluorinated carboxylic acids, while reduction can produce partially fluorinated compounds.
Scientific Research Applications
4-(Trifluoromethyl)hexafluoropent-2-enoic acid has several applications in scientific research, including:
Chemistry: It is used as a building block for the synthesis of more complex fluorinated compounds.
Biology: The compound’s unique properties make it useful in studying the effects of fluorination on biological systems.
Medicine: It is investigated for potential use in pharmaceuticals due to its stability and bioactivity.
Industry: The compound is used in the production of specialty chemicals and materials with enhanced properties, such as increased resistance to heat and chemicals.
Comparison with Similar Compounds
Perfluoro(4-methylpent-2-enoic acid): Similar in structure but with different fluorination patterns.
Hexafluoroisopropylidene bisphenol: Another highly fluorinated compound with distinct properties.
Uniqueness: 4-(Trifluoromethyl)hexafluoropent-2-enoic acid is unique due to the combination of trifluoromethyl and hexafluoro groups, which impart distinct chemical and physical properties. This makes it particularly valuable in applications requiring high stability and resistance to harsh conditions.
Biological Activity
Perfluoro(4-methylpent-2-enoic acid), commonly referred to as PFMeUPA, is a perfluorinated compound (PFAS) that has garnered attention due to its environmental persistence and potential biological activity. This article explores the biological activity of PFMeUPA, focusing on its microbial degradation pathways, mechanisms, and implications for bioremediation.
Overview of PFMeUPA
PFMeUPA is characterized by its unique structure, featuring a perfluorinated carbon chain with a double bond. This structure contributes to its stability and resistance to conventional degradation methods. The compound's formula is , indicating it contains multiple fluorinated carbon atoms, which typically confer high resistance to biodegradation.
Microbial Degradation Pathways
Recent studies have highlighted the potential for microbial communities to degrade PFMeUPA through reductive defluorination processes. Notably, specific anaerobic bacteria have shown capability in transforming this compound into less harmful substances:
- Anaerobic Microbial Communities : Research has demonstrated that certain anaerobic microbial communities can completely degrade PFMeUPA within extended incubation periods. For instance, one study reported complete degradation over 130 days using a consortium of microbes that included Acetobacterium bakii, which achieved significant biotransformation in just three weeks when grown on fructose .
- Key Microbial Players : The involvement of diverse microorganisms such as methanogens and homoacetogens has been noted in the degradation process. These microbes utilize various substrates (e.g., lactate, fructose) to facilitate the breakdown of PFAS compounds .
The degradation of PFMeUPA involves several biochemical mechanisms:
- Reductive Defluorination : This process is catalyzed by enzymes that facilitate the removal of fluorine atoms from the carbon chain. Studies indicate that an electron bifurcating flavin-iron–sulfur enzyme system plays a crucial role in this reaction . The precise fit of PFMeUPA in the active site of these enzymes suggests a highly specialized interaction that enhances degradation efficiency.
- Fluoride Management : A significant challenge in the biodegradation of PFAS is managing fluoride toxicity resulting from defluorination. Successful microbial strains often possess systems that export fluoride ions, mitigating their toxic effects on cell viability .
Case Study 1: Degradation by Acetobacterium bakii
In a controlled laboratory setting, Acetobacterium bakii was tested for its ability to degrade PFMeUPA. The results showed:
- Complete transformation of approximately 90 µM PFMeUPA within three weeks.
- The release of fluoride ions at a nearly stoichiometric ratio to the amount of PFMeUPA degraded.
- Identification of transformation products via mass spectrometry and NMR spectroscopy confirmed the specificity and efficiency of the microbial action .
Case Study 2: Community Dynamics
Another study investigated an anaerobic community enriched on lactate, revealing:
- A gradual decline in PFMeUPA concentration over 130 days.
- The community's ability to adapt and utilize different carbon sources while effectively degrading PFAS compounds.
- Insights into the metabolic pathways involved, suggesting potential avenues for enhancing bioremediation strategies through community engineering .
Implications for Bioremediation
The findings regarding PFMeUPA's biodegradability underscore its potential for bioremediation applications. Key implications include:
- Microbial Remediation Strategies : Utilizing specific microbial strains capable of degrading PFAS can be an effective strategy for remediating contaminated sites.
- Engineered Microbial Communities : Developing tailored microbial consortia that optimize degradation pathways could enhance efficiency and reduce remediation timeframes.
- Environmental Monitoring : Continuous monitoring and assessment of microbial activity in contaminated environments will be essential to evaluate remediation success and adapt strategies accordingly.
Properties
IUPAC Name |
(E)-2,3,4,5,5,5-hexafluoro-4-(trifluoromethyl)pent-2-enoic acid | |
---|---|---|
Source | PubChem | |
URL | https://pubchem.ncbi.nlm.nih.gov | |
Description | Data deposited in or computed by PubChem | |
InChI |
InChI=1S/C6HF9O2/c7-1(3(16)17)2(8)4(9,5(10,11)12)6(13,14)15/h(H,16,17)/b2-1+ | |
Source | PubChem | |
URL | https://pubchem.ncbi.nlm.nih.gov | |
Description | Data deposited in or computed by PubChem | |
InChI Key |
TWDHAMRDYDLSPH-OWOJBTEDSA-N | |
Source | PubChem | |
URL | https://pubchem.ncbi.nlm.nih.gov | |
Description | Data deposited in or computed by PubChem | |
Canonical SMILES |
C(=C(C(C(F)(F)F)(C(F)(F)F)F)F)(C(=O)O)F | |
Source | PubChem | |
URL | https://pubchem.ncbi.nlm.nih.gov | |
Description | Data deposited in or computed by PubChem | |
Isomeric SMILES |
C(=C(/C(C(F)(F)F)(C(F)(F)F)F)\F)(\C(=O)O)/F | |
Source | PubChem | |
URL | https://pubchem.ncbi.nlm.nih.gov | |
Description | Data deposited in or computed by PubChem | |
Molecular Formula |
C6HF9O2 | |
Source | PubChem | |
URL | https://pubchem.ncbi.nlm.nih.gov | |
Description | Data deposited in or computed by PubChem | |
DSSTOX Substance ID |
DTXSID50397558 | |
Record name | 3-(Perfluoroisopropyl)-(2E)-difluoropropenoic acid | |
Source | EPA DSSTox | |
URL | https://comptox.epa.gov/dashboard/DTXSID50397558 | |
Description | DSSTox provides a high quality public chemistry resource for supporting improved predictive toxicology. | |
Molecular Weight |
276.06 g/mol | |
Source | PubChem | |
URL | https://pubchem.ncbi.nlm.nih.gov | |
Description | Data deposited in or computed by PubChem | |
CAS No. |
103229-89-6 | |
Record name | 3-(Perfluoroisopropyl)-(2E)-difluoropropenoic acid | |
Source | EPA DSSTox | |
URL | https://comptox.epa.gov/dashboard/DTXSID50397558 | |
Description | DSSTox provides a high quality public chemistry resource for supporting improved predictive toxicology. | |
Record name | Perfluoro(4-methylpent-2-enoic acid) (trans) | |
Source | European Chemicals Agency (ECHA) | |
URL | https://echa.europa.eu/information-on-chemicals | |
Description | The European Chemicals Agency (ECHA) is an agency of the European Union which is the driving force among regulatory authorities in implementing the EU's groundbreaking chemicals legislation for the benefit of human health and the environment as well as for innovation and competitiveness. | |
Explanation | Use of the information, documents and data from the ECHA website is subject to the terms and conditions of this Legal Notice, and subject to other binding limitations provided for under applicable law, the information, documents and data made available on the ECHA website may be reproduced, distributed and/or used, totally or in part, for non-commercial purposes provided that ECHA is acknowledged as the source: "Source: European Chemicals Agency, http://echa.europa.eu/". Such acknowledgement must be included in each copy of the material. ECHA permits and encourages organisations and individuals to create links to the ECHA website under the following cumulative conditions: Links can only be made to webpages that provide a link to the Legal Notice page. | |
Retrosynthesis Analysis
AI-Powered Synthesis Planning: Our tool employs the Template_relevance Pistachio, Template_relevance Bkms_metabolic, Template_relevance Pistachio_ringbreaker, Template_relevance Reaxys, Template_relevance Reaxys_biocatalysis model, leveraging a vast database of chemical reactions to predict feasible synthetic routes.
One-Step Synthesis Focus: Specifically designed for one-step synthesis, it provides concise and direct routes for your target compounds, streamlining the synthesis process.
Accurate Predictions: Utilizing the extensive PISTACHIO, BKMS_METABOLIC, PISTACHIO_RINGBREAKER, REAXYS, REAXYS_BIOCATALYSIS database, our tool offers high-accuracy predictions, reflecting the latest in chemical research and data.
Strategy Settings
Precursor scoring | Relevance Heuristic |
---|---|
Min. plausibility | 0.01 |
Model | Template_relevance |
Template Set | Pistachio/Bkms_metabolic/Pistachio_ringbreaker/Reaxys/Reaxys_biocatalysis |
Top-N result to add to graph | 6 |
Feasible Synthetic Routes
Q1: Can microorganisms break down PFMeUPA, despite its strong carbon-fluorine bonds?
A: Yes, recent research has demonstrated that microbial communities capable of organohalide-respiration can cleave the carbon-fluorine (C-F) bonds in PFMeUPA. This groundbreaking discovery shows that reductive defluorination can occur in this perfluorinated compound, challenging the previous belief that such biodegradation was impossible. []
Q2: How does the structure of PFMeUPA influence its susceptibility to microbial defluorination?
A: The presence of a double bond (C=C) in the PFMeUPA structure appears to play a crucial role in its susceptibility to microbial reductive defluorination. Research suggests that this unsaturated bond facilitates the cleavage of C-F bonds, potentially by weakening them and making them more accessible to microbial attack. [, ]
Q3: What are the potential environmental implications of microbial PFMeUPA degradation?
A: The discovery of microbial PFMeUPA degradation through reductive defluorination offers a promising avenue for developing bioremediation strategies for PFAS-contaminated environments. This finding expands our understanding of PFAS environmental fate and suggests that naturally occurring microbial processes could contribute to the attenuation of these persistent pollutants. []
Disclaimer and Information on In-Vitro Research Products
Please be aware that all articles and product information presented on BenchChem are intended solely for informational purposes. The products available for purchase on BenchChem are specifically designed for in-vitro studies, which are conducted outside of living organisms. In-vitro studies, derived from the Latin term "in glass," involve experiments performed in controlled laboratory settings using cells or tissues. It is important to note that these products are not categorized as medicines or drugs, and they have not received approval from the FDA for the prevention, treatment, or cure of any medical condition, ailment, or disease. We must emphasize that any form of bodily introduction of these products into humans or animals is strictly prohibited by law. It is essential to adhere to these guidelines to ensure compliance with legal and ethical standards in research and experimentation.