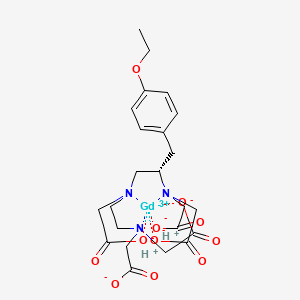
Gadoxetic acid
- Click on QUICK INQUIRY to receive a quote from our team of experts.
- With the quality product at a COMPETITIVE price, you can focus more on your research.
Overview
Description
Gadoxetic acid (gadolinium-ethoxybenzyl-diethylenetriamine pentaacetic acid, Gd-EOB-DTPA) is a hepatocyte-specific magnetic resonance imaging (MRI) contrast agent widely used for liver imaging. It is actively transported into hepatocytes via the organic anion-transporting polypeptide 1 (OATP1) and excreted through the multidrug resistance-associated protein 2 (MRP2) into bile ducts, enabling dual-phase imaging: dynamic vascular phases (arterial, portal venous, and delayed phases) and hepatobiliary phase (HBP) imaging (20–60 minutes post-injection) . Approximately 50% of the injected dose is taken up by hepatocytes, leading to pronounced parenchymal enhancement in normal liver tissue, while impaired uptake occurs in fibrosis or cirrhosis due to reduced OATP1/MRP2 expression . This compound-enhanced MRI (GA-MRI) provides superior soft-tissue contrast and functional insights, making it invaluable for detecting hepatocellular carcinoma (HCC), assessing liver fibrosis, and evaluating biliary excretion .
Preparation Methods
Key Intermediates in Gadoxetic Acid Synthesis
The synthesis of this compound hinges on the production of its tricarboxylate intermediate (compound I). This intermediate features a stereospecific S-configuration at the 4-position, an ethoxybenzyl group, and three carboxylmethyl arms attached to a triazaundecanedioic acid backbone. Early methods suffered from low yields (50–60%) due to steric hindrance during tert-butyl ester hydrolysis . Modern approaches bypass multi-step protection-deprotection sequences, instead using sodium chloroacetate or bromoacetate for direct carboxylation .
Synthesis of Compound I
One-Step Alkylation-Carboxylation
A breakthrough method involves reacting S-1-(4-hydroxyphenyl)-3-aza-pentane-1,5-diamine (compound III) with sodium bromoacetate or chloroacetate in aqueous sodium hydroxide :
Reaction Conditions
-
Molar Ratio : 1:7 (compound III : sodium bromoacetate)
-
Temperature : Reflux (100–110°C)
-
Duration : 20–40 hours
-
Yield : 73.8–77.4%
This method eliminates tert-butyl ester intermediates, reducing steric hindrance and side reactions. Sodium hydroxide facilitates deprotonation, enhancing nucleophilic substitution at the amine centers.
Comparative Analysis of Carboxylation Agents
Agent | Molar Excess | Reaction Time (h) | Yield (%) | Purity (%) |
---|---|---|---|---|
Sodium Bromoacetate | 7x | 20 | 73.8 | 98.0 |
Sodium Chloroacetate | 10x | 40 | 77.4 | 98.7 |
Sodium chloroacetate’s higher reactivity offsets its slower kinetics, achieving superior yields despite longer reaction times .
Purification Techniques
Crude compound I often contains unreacted starting materials, sodium salts, and dimers. Patent CN109232279B details a resin-based purification process :
Macroporous Adsorption Resin Protocol
-
Dissolution : 1 kg crude compound I in 2.8 L water.
-
Adsorption : LX-18 resin (280 mL), 25°C, 30-minute contact.
-
Elution : 30% methanol in water at 1 L/h flow rate.
-
Concentration : 50°C under reduced pressure.
Outcomes
Resin selection is critical:
-
LS-620 : Best for hydrochloride salts (100% purity).
-
LX-18 : Ideal for free bases (99.8% purity).
Industrial-Scale Production Considerations
Environmental and Economic Advantages
-
Solvent-Free Reactions : Aqueous conditions reduce volatile organic compound (VOC) emissions .
-
Recyclable Resins : Macroporous resins withstand >100 cycles without efficiency loss .
-
Cost Savings : Sodium chloroacetate ($12/kg) vs. bromoacetate ($45/kg) .
Scalability Challenges
Chemical Reactions Analysis
Types of Reactions
Gadoxetic acid primarily undergoes complexation reactions due to the presence of multiple carboxylate and amine groups in the EOB-DTPA ligand. These groups coordinate with the gadolinium ion to form a stable complex.
Common Reagents and Conditions
Reagents: Diethylenetriaminepentaacetic acid, ethoxybenzyl chloride, gadolinium chloride.
Major Products
The major product of these reactions is gadoxetate disodium, a stable gadolinium complex used as a contrast agent in MRI .
Scientific Research Applications
Diagnostic Applications
Hepatobiliary Imaging
Gadoxetic acid is primarily used in MRI to enhance the visualization of liver lesions and the biliary system. It is absorbed by hepatocytes and excreted into bile ducts, allowing for clear differentiation between normal and abnormal liver tissues. This property is particularly beneficial in diagnosing conditions such as hepatocellular carcinoma (HCC) and metastatic liver disease.
- Detection of Focal Liver Lesions : this compound-enhanced MRI has shown high sensitivity and specificity in identifying focal liver lesions. Studies indicate that it provides critical information during the hepatobiliary phase, which aids in distinguishing between benign and malignant lesions .
- Staging of Hepatocellular Carcinoma : The agent is instrumental in accurately staging HCC before and after treatment. It allows for precise assessment of tumor size, vascular involvement, and metastasis, which are crucial for treatment planning .
- Monitoring Treatment Response : this compound-enhanced MRI can evaluate how well tumors respond to systemic therapies. Changes in imaging characteristics post-treatment can provide insights into the effectiveness of therapies .
Evaluating Liver Function
Functional Assessment
this compound's unique uptake mechanism allows it to serve as a tool for assessing liver function. Its distribution patterns can reflect the functional status of hepatocytes.
- Liver Fibrosis Staging : Recent studies have employed this compound-enhanced imaging to stage liver fibrosis using radiomics analysis. This method correlates imaging features with histological findings, providing a non-invasive alternative to biopsy .
- Biliary Function Assessment : The agent's ability to visualize biliary excretion makes it valuable for evaluating biliary diseases, including cholestasis and biliary obstructions. It helps assess the patency of bile ducts and can identify pathological changes .
Advanced Imaging Techniques
Integration with Artificial Intelligence
The incorporation of artificial intelligence (AI) into this compound-enhanced MRI is an emerging field that promises to enhance diagnostic accuracy further. AI algorithms can analyze complex imaging data to identify patterns that may not be visible to the human eye, improving the detection rates of liver lesions and aiding in prognosis predictions .
Case Studies
Several case studies highlight the utility of this compound in clinical practice:
- Case Study on Oxaliplatin-Induced Hepatic Sinusoidal Obstruction Syndrome (HSOS) : A study demonstrated that this compound-enhanced MRI effectively detected HSOS in patients undergoing chemotherapy for metastatic colon cancer. The imaging findings correlated with liver function tests, illustrating its diagnostic value in specific clinical scenarios .
- Assessment of Liver Metastases : In a cohort study involving patients with colorectal liver metastases, this compound-enhanced MRI provided superior staging information compared to conventional imaging techniques. The ability to visualize both vascular and hepatobiliary phases was crucial for accurate treatment planning .
Mechanism of Action
Gadoxetic acid disodium is a paramagnetic compound that develops a magnetic moment when placed in a magnetic field. This property enhances the T1 signal intensity in MRI, making it easier to visualize internal structures. The compound is selectively taken up by hepatocytes and excreted into the bile, providing a clear contrast between healthy and diseased liver tissue .
Comparison with Similar Compounds
Gadoxetic acid is compared below with other MRI contrast agents and imaging modalities in terms of pharmacokinetics, diagnostic performance, and clinical utility.
Pharmacokinetic and Physicochemical Properties
Parameter | This compound | Gadopentetate Dimeglumine | Gadobenate Dimeglumine | Iminodiacetic Acid (IDA) SPECT |
---|---|---|---|---|
Hepatocyte Uptake | 50% via OATP1 | None (extracellular agent) | Partial (3–5%) via OATP1 | Yes (hepatocyte uptake) |
Biliary Excretion | 50% via MRP2 | None | Minimal (~5%) | Yes |
Renal Excretion | 50% | 100% | ~95% | None |
Relaxivity (T1, mmol⁻¹s⁻¹) | 6.9 (blood), 16.6 (liver) | 4.1–4.3 | 6.0–6.5 | N/A |
HBP Timing | 20–60 minutes | N/A | 60–120 minutes | N/A |
Key Findings :
- This compound has higher hepatocyte uptake and faster biliary excretion than gadobenate dimeglumine, enabling earlier HBP imaging .
- Its liver-specific relaxivity (16.6 mmol⁻¹s⁻¹) enhances parenchymal contrast but is lower than extracellular agents in blood .
- IDA SPECT shares hepatocyte uptake but lacks the spatial resolution of GA-MRI .
Diagnostic Performance in Hepatocellular Carcinoma (HCC)
Key Findings :
- GA-MRI’s HBP improves lesion conspicuity and sensitivity for small HCCs (<2 cm) compared to extracellular agents .
- Corona enhancement on GA-MRI can substitute for capsule appearance in HCC diagnosis .
Liver Function and Fibrosis Assessment
Parameter | This compound | Extracellular Agents | Transient Elastography |
---|---|---|---|
Fibrosis Detection | High (Ishak scores 1–3) | Low | High (cirrhosis only) |
Functional Quantification | Yes (RE, T1 mapping) | No | No |
Biliary Excretion | Yes (qualitative/quantitative) | No | No |
Key Findings :
- GA-MRI detects early fibrosis (Ishak 1–2) via reduced T1 signal enhancement ratios, outperforming blood tests (e.g., ALT, AST) .
- Absent biliary excretion of this compound in HBP correlates with advanced PSC and worse MELD scores .
Clinical Guidelines
- APASL Guidelines : Recommend GA-MRI over extracellular contrast MRI for HCC .
- LI-RADS v2018 : GA-MRI allows LR-5 categorization without arterial phase hyperenhancement if HBP hypointensity and ancillary features (e.g., corona enhancement) are present .
Limitations and Challenges
- False Positives: Benign lesions (e.g., focal nodular hyperplasia) may show HBP hyperintensity .
- Timing Variability: HBP image acquisition timing (15–60 minutes) affects CNR and diagnostic consistency .
- Cost and Accessibility : GA-MRI is more expensive than CT or extracellular agent-based MRI .
Q & A
Basic Research Questions
Q. What are the critical considerations for optimizing dynamic and hepatobiliary phase imaging protocols in gadoxetic acid-enhanced MRI studies?
- Methodological Answer: The arterial, portal venous, and hepatobiliary phases must be timed precisely to capture vascular perfusion and hepatocyte uptake. For dynamic phases, use a test bolus or fixed delay (e.g., 20–30 sec for arterial phase). For the hepatobiliary phase (HBP), image acquisition at 20 minutes post-injection is standard, but delayed acquisitions (30–60 minutes) may improve visualization in chronic liver disease . Ensure consistent breath-holding protocols to minimize motion artifacts, and validate timing with patient-specific factors (e.g., cardiac output, liver function) .
Q. How can researchers address transient motion artifacts during this compound-enhanced MRI?
- Methodological Answer: Transient motion artifacts (occurring in ~40% of patients) are linked to breath-hold failure rather than dyspnea. Mitigation strategies include:
- Respiratory gating techniques (e.g., free-breathing golden-angle radial sparse parallel [GRASP] MRI) to reconstruct motion-free images .
- Shortening acquisition times (e.g., 6-second temporal resolution) to reduce artifact severity .
- Pre-screening for high-risk patients (e.g., males, higher BMI) and training patients on breath-holding protocols .
Q. What statistical methods are recommended for analyzing this compound-enhanced MRI data in hepatocellular carcinoma (HCC) prognosis studies?
- Methodological Answer: Use Kaplan-Meier survival analysis with log-rank tests to compare groups (e.g., high vs. low this compound uptake). For multivariate analysis, Cox proportional hazard models should include covariates like Child-Pugh class, ALBI grade, and tumor size. Ensure adjustment for confounding variables (e.g., treatment modality) and validate model assumptions (proportional hazards) .
Q. How does this compound-enhanced MRI compare to multidetector CT (MDCT) in detecting liver metastases?
- Methodological Answer: In comparative studies, this compound MRI shows superior sensitivity (96.4% vs. 70.9% for MDCT) for small metastases (0.3–1.0 cm). Use blinded, multi-reader designs with histopathology or follow-up imaging as reference standards. Report interobserver agreement via kappa statistics and address discordant results through consensus review .
Advanced Research Questions
Q. How can radiomic features derived from this compound-enhanced MRI predict treatment response in chronic liver disease?
- Methodological Answer: Extract texture features (e.g., entropy, heterogeneity) from HBP images using software like PyRadiomics. Apply machine learning models (e.g., random forests) to correlate features with histopathological fibrosis scores (Ishak or METAVIR) or serum biomarkers (e.g., hyaluronic acid). Validate models in independent cohorts and account for variations in MRI scanner protocols .
Q. What molecular mechanisms explain the correlation between this compound uptake and β-catenin mutations in HCC?
- Methodological Answer: β-catenin-activated HCCs exhibit upregulated OATP1B1/3 expression, enhancing this compound uptake. Conversely, MRP3 overexpression (linked to Wnt/β-catenin signaling) increases biliary excretion, causing washout. Validate these mechanisms via immunohistochemical staining of transporter proteins and correlate with quantitative HBP signal intensity ratios .
Q. How can this compound-enhanced MRI quantify regional liver function in primary sclerosing cholangitis (PSC)?
- Methodological Answer: Calculate relative enhancement (RE) as RE=(SIHBP−SIpre-contrast)/SIpre-contrast. Use linear mixed models to assess longitudinal changes in RE and biliary excretion, correlating with MELD scores or ALP levels. ROC analysis can identify RE cutoffs (e.g., 0.43 for biliary excretion; sensitivity 85.9%) to stratify disease severity .
Q. What methodologies are effective for assessing gadolinium retention in the brain after repeated this compound administration?
- Methodological Answer: Measure T1-weighted signal intensity (SI) ratios (e.g., dentate nucleus/middle cerebellar peduncle [DN/MCP]) in patients with ≥10 administrations. Use cross-sectional studies with age-matched controls and non-parametric tests (e.g., Kruskal-Wallis). Correlate SI ratios with cumulative this compound dose via Spearman’s rank correlation .
Q. How can artificial intelligence (AI) improve the detection of small HCCs using combined this compound-enhanced and diffusion-weighted MRI?
- Methodological Answer: Train convolutional neural networks (CNNs) on multi-sequence datasets (arterial phase, HBP, ADC maps). Use fusion algorithms to integrate features, achieving higher sensitivity (91–93%) than single-modality analysis. Validate with surgical pathology and report performance via area under the ROC curve (AUC) .
Q. What experimental designs are optimal for evaluating this compound as a surrogate biomarker of liver fibrosis in preclinical models?
- Methodological Answer: In rodent models (e.g., TAA-induced fibrosis), use dynamic contrast-enhanced MRI to calculate the hepatic uptake rate (k₁) via a linearized two-compartment model. Correlate k₁ with histopathology (collagen proportion area) and transporter expression (OATP1, MRP2) using qPCR or Western blot .
Properties
Key on ui mechanism of action |
Gadoxetate disodium is a paramagnetic compound and develops a magnetic moment when placed in a magnetic field. The relatively large magnetic moment produced by gadoxetate disodium results in a local magnetic field, yielding enhanced relaxation rates (shortening of relaxation times) of water protons in the vicinity of the paramagnetic agent, which leads to an increase in signal intensity (brightening) of blood and tissue. In MRI, visualization of normal and pathological tissue depends in part on variations in the radiofrequency signal intensity that occur with 1) differences in proton density; 2) differences of the spin-lattice or longitudinal relaxation times (T1); and 3) differences in the spin-spin or transverse relaxation time (T2). When placed in a magnetic field, gadoxetate disodium decreases the T1 and T2 relaxation time in target tissue. At the recommended dose, the effect is observed with the greatest sensitivity in T1-weighted MR sequences. |
---|---|
CAS No. |
135326-11-3 |
Molecular Formula |
C23H30GdN3O11 |
Molecular Weight |
681.7 g/mol |
IUPAC Name |
2-[2-[bis(carboxylatomethyl)amino]ethyl-[(2S)-2-[bis(carboxymethyl)amino]-3-(4-ethoxyphenyl)propyl]amino]acetate;gadolinium(3+) |
InChI |
InChI=1S/C23H33N3O11.Gd/c1-2-37-18-5-3-16(4-6-18)9-17(26(14-22(33)34)15-23(35)36)10-24(11-19(27)28)7-8-25(12-20(29)30)13-21(31)32;/h3-6,17H,2,7-15H2,1H3,(H,27,28)(H,29,30)(H,31,32)(H,33,34)(H,35,36);/q;+3/p-3/t17-;/m0./s1 |
InChI Key |
PCZHWPSNPWAQNF-LMOVPXPDSA-K |
SMILES |
[H+].[H+].CCOC1=CC=C(C=C1)CC(CN(CCN(CC(=O)[O-])CC(=O)[O-])CC(=O)[O-])N(CC(=O)[O-])CC(=O)[O-].[Gd+3] |
Isomeric SMILES |
CCOC1=CC=C(C=C1)C[C@@H](CN(CCN(CC(=O)[O-])CC(=O)[O-])CC(=O)[O-])N(CC(=O)O)CC(=O)O.[Gd+3] |
Canonical SMILES |
CCOC1=CC=C(C=C1)CC(CN(CCN(CC(=O)[O-])CC(=O)[O-])CC(=O)[O-])N(CC(=O)O)CC(=O)O.[Gd+3] |
Origin of Product |
United States |
Disclaimer and Information on In-Vitro Research Products
Please be aware that all articles and product information presented on BenchChem are intended solely for informational purposes. The products available for purchase on BenchChem are specifically designed for in-vitro studies, which are conducted outside of living organisms. In-vitro studies, derived from the Latin term "in glass," involve experiments performed in controlled laboratory settings using cells or tissues. It is important to note that these products are not categorized as medicines or drugs, and they have not received approval from the FDA for the prevention, treatment, or cure of any medical condition, ailment, or disease. We must emphasize that any form of bodily introduction of these products into humans or animals is strictly prohibited by law. It is essential to adhere to these guidelines to ensure compliance with legal and ethical standards in research and experimentation.