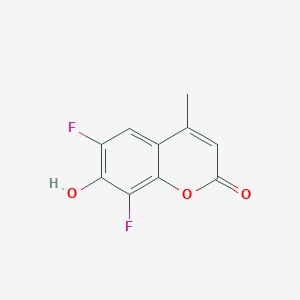
6,8-Difluoro-7-hydroxy-4-methylcoumarin
Overview
Description
6,8-Difluoro-7-hydroxy-4-methylcoumarin is a fluorophore, which means it is a compound that can re-emit light upon light excitation. This compound is known for its blue fluorescence and is used in various biochemical applications, including as a reference standard for ether, ester, and phosphate substrates .
Mechanism of Action
Target of Action
6,8-Difluoro-7-hydroxy-4-methylcoumarin, also known as DiFMU, is primarily used as a fluorogenic substrate . It has been used for the diagnostic detection of natural killer cell-activity . It has been conjugated to streptavidin or secondary antibodies for flow cytometry and used to label bacteria for multi-color fluorescence in situ hybridization (FISH) .
Mode of Action
DiFMU can be used as a reference standard for ether, ester, and phosphate substrates derived from this blue-fluorescent dye . It interacts with its targets by binding to them and emitting fluorescence, which can be detected and measured .
Biochemical Pathways
It has been used in the study of bacterial two-component sensor kinases, which possess a histidine-containing phosphotransfer (hpt) domain to carry out a multistep phosphotransferring reaction to a cognate response regulator .
Result of Action
The primary result of DiFMU’s action is the emission of fluorescence when it interacts with its targets . This fluorescence can be used to track and measure the activity of the targets, providing valuable information in biological research and diagnostics .
Action Environment
The action, efficacy, and stability of DiFMU can be influenced by various environmental factors. For instance, the pH of the environment can affect the optimal operation of the targets of DiFMU . Additionally, factors such as temperature and light exposure can influence the stability and fluorescence of DiFMU .
Biochemical Analysis
Biochemical Properties
6,8-Difluoro-7-hydroxy-4-methylcoumarin plays a significant role in biochemical reactions due to its ability to act as a fluorogenic substrate. It interacts with enzymes such as aryl sulfatases and organophosphatases, where it undergoes hydrolysis to produce a fluorescent product . This interaction is crucial for the continuous assaying of these enzymes, providing insights into their activity and function. Additionally, this compound has been conjugated to streptavidin or secondary antibodies for flow cytometry and used to label bacteria for multi-color fluorescence in situ hybridization (FISH) .
Cellular Effects
This compound influences various cellular processes by acting as a fluorescent probe. It has been shown to label cells effectively, aiding in the visualization of cellular structures and functions . This compound can impact cell signaling pathways, gene expression, and cellular metabolism by providing a means to track and analyze these processes in real-time. Its use in flow cytometry and FISH highlights its ability to interact with cellular components and provide valuable data on cell function .
Molecular Mechanism
At the molecular level, this compound exerts its effects through specific binding interactions with biomolecules. It reacts efficiently with primary amines, forming stable, covalent amide bonds . This property allows it to be used in labeling proteins and other biomolecules, facilitating the study of their interactions and functions. The compound’s ability to act as a fluorogenic substrate for enzymes like aryl sulfatases and organophosphatases further underscores its role in biochemical assays .
Temporal Effects in Laboratory Settings
In laboratory settings, the effects of this compound can change over time. The compound is stable when stored at room temperature and protected from light . Its long-term effects on cellular function have been observed in both in vitro and in vivo studies. These studies indicate that the compound maintains its fluorescent properties over extended periods, making it a reliable tool for long-term experiments .
Dosage Effects in Animal Models
The effects of this compound vary with different dosages in animal models. At lower doses, the compound effectively labels cells and tissues without causing significant toxicity . At higher doses, there may be threshold effects, including potential toxicity or adverse reactions. These findings highlight the importance of optimizing dosage to achieve the desired effects while minimizing any negative impact .
Metabolic Pathways
This compound is involved in metabolic pathways that include interactions with enzymes and cofactors. It acts as a substrate for enzymes like aryl sulfatases and organophosphatases, undergoing hydrolysis to produce fluorescent products . These interactions are essential for studying metabolic flux and metabolite levels, providing insights into the compound’s role in cellular metabolism .
Transport and Distribution
Within cells and tissues, this compound is transported and distributed through interactions with transporters and binding proteins. Its solubility in solvents like methanol, DMSO, and dimethyl formamide facilitates its use in various experimental setups . The compound’s localization and accumulation within specific cellular compartments are influenced by these interactions, affecting its overall activity and function .
Subcellular Localization
The subcellular localization of this compound is determined by targeting signals and post-translational modifications. These factors direct the compound to specific compartments or organelles, where it exerts its effects . Understanding its localization is crucial for interpreting its activity and function within the cellular context .
Preparation Methods
The synthesis of 6,8-Difluoro-7-hydroxy-4-methylcoumarin typically involves the following steps:
Starting Materials: The synthesis begins with the appropriate substituted phenol and ethyl acetoacetate.
Reaction Conditions: The reaction is carried out in the presence of a base, such as sodium ethoxide, and a solvent like ethanol.
Cyclization: The intermediate product undergoes cyclization to form the coumarin structure.
Industrial production methods may vary, but they generally follow similar synthetic routes with optimizations for large-scale production.
Chemical Reactions Analysis
6,8-Difluoro-7-hydroxy-4-methylcoumarin undergoes various chemical reactions, including:
Oxidation: This compound can be oxidized to form quinones.
Reduction: Reduction reactions can convert it to dihydro derivatives.
Substitution: Halogen atoms can be substituted with other functional groups using appropriate reagents and conditions.
Common reagents used in these reactions include oxidizing agents like potassium permanganate, reducing agents like sodium borohydride, and nucleophiles for substitution reactions .
Scientific Research Applications
6,8-Difluoro-7-hydroxy-4-methylcoumarin has several scientific research applications:
Chemistry: Used as a fluorescent probe in various chemical assays.
Biology: Employed in fluorescence microscopy and flow cytometry to label and visualize biological samples.
Medicine: Utilized in diagnostic assays to detect specific biomolecules.
Industry: Applied in the development of fluorescent dyes and sensors.
Comparison with Similar Compounds
6,8-Difluoro-7-hydroxy-4-methylcoumarin can be compared with other similar compounds such as:
7-Hydroxy-4-methylcoumarin: Lacks the fluorine atoms, resulting in different fluorescence properties.
6,7-Dihydroxy-4-methylcoumarin: Contains additional hydroxyl groups, affecting its reactivity and fluorescence.
4-Methylumbelliferone: A widely used fluorophore with different excitation and emission wavelengths.
The uniqueness of this compound lies in its specific fluorescence properties, making it suitable for applications requiring blue fluorescence .
Properties
IUPAC Name |
6,8-difluoro-7-hydroxy-4-methylchromen-2-one | |
---|---|---|
Source | PubChem | |
URL | https://pubchem.ncbi.nlm.nih.gov | |
Description | Data deposited in or computed by PubChem | |
InChI |
InChI=1S/C10H6F2O3/c1-4-2-7(13)15-10-5(4)3-6(11)9(14)8(10)12/h2-3,14H,1H3 | |
Source | PubChem | |
URL | https://pubchem.ncbi.nlm.nih.gov | |
Description | Data deposited in or computed by PubChem | |
InChI Key |
LLENVBUPWUQAGL-UHFFFAOYSA-N | |
Source | PubChem | |
URL | https://pubchem.ncbi.nlm.nih.gov | |
Description | Data deposited in or computed by PubChem | |
Canonical SMILES |
CC1=CC(=O)OC2=C(C(=C(C=C12)F)O)F | |
Source | PubChem | |
URL | https://pubchem.ncbi.nlm.nih.gov | |
Description | Data deposited in or computed by PubChem | |
Molecular Formula |
C10H6F2O3 | |
Source | PubChem | |
URL | https://pubchem.ncbi.nlm.nih.gov | |
Description | Data deposited in or computed by PubChem | |
DSSTOX Substance ID |
DTXSID901318263 | |
Record name | Marina Blue | |
Source | EPA DSSTox | |
URL | https://comptox.epa.gov/dashboard/DTXSID901318263 | |
Description | DSSTox provides a high quality public chemistry resource for supporting improved predictive toxicology. | |
Molecular Weight |
212.15 g/mol | |
Source | PubChem | |
URL | https://pubchem.ncbi.nlm.nih.gov | |
Description | Data deposited in or computed by PubChem | |
CAS No. |
215868-23-8 | |
Record name | Marina Blue | |
Source | CAS Common Chemistry | |
URL | https://commonchemistry.cas.org/detail?cas_rn=215868-23-8 | |
Description | CAS Common Chemistry is an open community resource for accessing chemical information. Nearly 500,000 chemical substances from CAS REGISTRY cover areas of community interest, including common and frequently regulated chemicals, and those relevant to high school and undergraduate chemistry classes. This chemical information, curated by our expert scientists, is provided in alignment with our mission as a division of the American Chemical Society. | |
Explanation | The data from CAS Common Chemistry is provided under a CC-BY-NC 4.0 license, unless otherwise stated. | |
Record name | Marina Blue | |
Source | EPA DSSTox | |
URL | https://comptox.epa.gov/dashboard/DTXSID901318263 | |
Description | DSSTox provides a high quality public chemistry resource for supporting improved predictive toxicology. | |
Synthesis routes and methods
Procedure details
Q1: What makes 6,8-Difluoro-7-hydroxy-4-methylcoumarin useful for cell imaging?
A1: this compound, also known as Marina Blue, exhibits blue fluorescence. This property makes it valuable for cell imaging applications. [] Researchers can conjugate Marina Blue to sugar derivatives, creating fluorescent probes. These probes can then be used to visualize and track cells, as well as molecules within cells. []
Q2: Are there specific applications for this compound in cancer research?
A2: Yes, research indicates the potential of this compound as a cancer imaging agent. Specifically, when conjugated to L-glucose, it can be used to visualize and potentially target cancerous cells. [] This application utilizes the molecule's fluorescent properties for improved precision in imaging cancerous cells.
Disclaimer and Information on In-Vitro Research Products
Please be aware that all articles and product information presented on BenchChem are intended solely for informational purposes. The products available for purchase on BenchChem are specifically designed for in-vitro studies, which are conducted outside of living organisms. In-vitro studies, derived from the Latin term "in glass," involve experiments performed in controlled laboratory settings using cells or tissues. It is important to note that these products are not categorized as medicines or drugs, and they have not received approval from the FDA for the prevention, treatment, or cure of any medical condition, ailment, or disease. We must emphasize that any form of bodily introduction of these products into humans or animals is strictly prohibited by law. It is essential to adhere to these guidelines to ensure compliance with legal and ethical standards in research and experimentation.