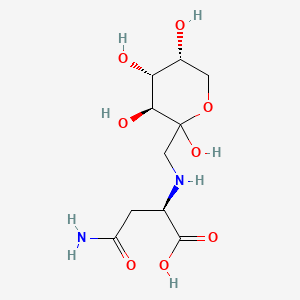
Fructose-asparagine
Overview
Description
Fructose-asparagine is a unique compound formed through the nonenzymatic condensation of glucose and asparagine. This compound is particularly significant in the context of food science and microbiology, as it serves as a primary nutrient for certain bacteria, such as Salmonella enterica .
Mechanism of Action
Target of Action
Fructose-asparagine (F-Asn) is a glycosylamine compound that is primarily utilized by Salmonella during inflammation of the intestine . The genetic locus that encodes the uptake capability in Salmonella is fra
, which includes five genes: fraR
(a regulator), fraB
(a this compound deglycase), fraD
(a sugar kinase), fraA
(a this compound transporter), and fraE
(a L-asparaginase) .
Mode of Action
The fra
locus in Salmonella encodes an uptake and utilization pathway specific for F-Asn . The fraE
gene encodes the periplasmic fructose-asparaginase FraE that removes ammonia from F-Asn to form fructose-aspartate (F-Asp) . Notably, mutations in fraB
cause the buildup of the toxic intermediate 6-phosphofructose-aspartate (6-P-F-Asp), which has a bacteriostatic effect on fraB
mutant cells .
Biochemical Pathways
The biochemical pathway for F-Asn utilization involves the conversion of F-Asn into 6-P-F-Asp, which accumulates in the absence of FraB . This accumulation is expected from the predicted pathway for F-Asn utilization . The buildup of 6-P-F-Asp has a bacteriostatic effect on fraB
mutant cells, making FraB a potential drug target .
Pharmacokinetics
It is known that f-asn is essential for salmonella’s fitness in the inflamed intestine . The fra
locus provides an advantage only if Salmonella can initiate inflammation and use tetrathionate as a terminal electron acceptor for anaerobic respiration .
Result of Action
The utilization of F-Asn by Salmonella during inflammation leads to a severe fitness defect in a Salmonella fra
mutant, suggesting that F-Asn is the primary nutrient utilized by Salmonella during inflammation . This system provides a valuable target for novel therapies .
Action Environment
The action of F-Asn is influenced by the environment within the inflamed intestine. Salmonella relies heavily on F-Asn during growth in the inflamed intestine . The fra
phenotype is lost in Salmonella SPI1 − SPI2 − or ttrA
mutants, respectively . This suggests that the action, efficacy, and stability of F-Asn are influenced by the inflammatory environment within the intestine .
Biochemical Analysis
Biochemical Properties
Fructose-asparagine plays a crucial role in biochemical reactions, particularly in the metabolism of certain bacteria. It serves as a source of carbon and nitrogen for Salmonella enterica serovar Typhimurium . The enzymes involved in the utilization of this compound include FraB (deglycase), FraD (kinase), and FraE (asparaginase) . FraB catalyzes the deglycation of this compound, FraD phosphorylates the resulting product, and FraE hydrolyzes the asparagine moiety. These interactions are essential for the bacteria to utilize this compound effectively.
Cellular Effects
This compound influences various cellular processes, particularly in bacteria. In Salmonella enterica, it has been shown to be bacteriostatic to fraB mutants, leading to the accumulation of a toxic metabolite, 6-phosphofructose-aspartate . This accumulation inhibits the growth of the bacteria, highlighting the importance of the FraB enzyme in detoxifying this intermediate. The presence of this compound in the gut can also affect the competition between different bacterial species, as some bacteria can utilize this compound while others cannot .
Molecular Mechanism
The molecular mechanism of this compound involves its metabolism through a specific pathway in bacteria. The initial step is the deglycation by FraB, followed by phosphorylation by FraD, and hydrolysis by FraE . The accumulation of 6-phosphofructose-aspartate in fraB mutants suggests that the absence of FraB leads to the buildup of this toxic intermediate, which inhibits bacterial growth . This pathway is unique to certain bacteria and represents a potential target for antimicrobial therapies.
Temporal Effects in Laboratory Settings
In laboratory settings, the effects of this compound can change over time. The stability and degradation of this compound are influenced by various factors, including the presence of specific enzymes and environmental conditions. Long-term studies have shown that the accumulation of toxic intermediates, such as 6-phosphofructose-aspartate, can have lasting effects on bacterial growth and metabolism . These findings underscore the importance of temporal dynamics in understanding the impact of this compound on cellular function.
Dosage Effects in Animal Models
The effects of this compound vary with different dosages in animal models. Studies have shown that low doses of this compound can be utilized effectively by bacteria, while high doses may lead to the accumulation of toxic intermediates and adverse effects on bacterial growth . These threshold effects highlight the importance of dosage in determining the impact of this compound on microbial metabolism and health.
Metabolic Pathways
This compound is involved in specific metabolic pathways in bacteria. The enzymes FraB, FraD, and FraE play key roles in its metabolism, converting this compound into usable forms of carbon and nitrogen . This pathway is unique to certain bacteria and represents a potential target for antimicrobial therapies. The interaction of this compound with these enzymes affects metabolic flux and the levels of various metabolites within the cell.
Transport and Distribution
The transport and distribution of this compound within cells and tissues involve specific transporters and binding proteins. In bacteria, the uptake of this compound is facilitated by transporters that recognize this compound and allow its entry into the cell . Once inside, this compound is metabolized through the specific pathway involving FraB, FraD, and FraE. The localization and accumulation of this compound within the cell are influenced by these transporters and the activity of the enzymes involved in its metabolism.
Subcellular Localization
The subcellular localization of this compound and its effects on activity or function are determined by targeting signals and post-translational modifications. In bacteria, this compound is localized within specific compartments where the enzymes FraB, FraD, and FraE are active . These enzymes direct this compound to the appropriate subcellular locations, ensuring its effective metabolism and utilization. The targeting signals and modifications that direct this compound to these compartments are essential for its function and impact on cellular processes.
Preparation Methods
Synthetic Routes and Reaction Conditions
Fructose-asparagine is synthesized through an Amadori rearrangement, which is a part of the Maillard reaction. This reaction involves the nonenzymatic condensation of the carbonyl group of reducing sugars (such as glucose) with the nucleophilic amino group of amino acids (such as asparagine). The initial step forms a Schiff base, which spontaneously rearranges to form a stable ketoamine .
Industrial Production Methods
In industrial settings, this compound can be produced by heating mixtures of glucose and asparagine under controlled conditions. The reaction parameters, such as pH, temperature, and solvent, are carefully monitored to optimize the yield of this compound .
Chemical Reactions Analysis
Types of Reactions
Fructose-asparagine undergoes several types of chemical reactions, including:
Maillard Reaction: This reaction occurs between reducing sugars and amino acids, leading to the formation of various compounds, including acrylamide.
Oxidation and Reduction: this compound can participate in redox reactions, although specific details on these reactions are less documented.
Common Reagents and Conditions
Reducing Sugars: Glucose is commonly used in the synthesis of this compound.
Amino Acids: Asparagine is the primary amino acid involved in the formation of this compound.
Major Products Formed
Scientific Research Applications
Fructose-asparagine has several scientific research applications:
Comparison with Similar Compounds
Similar Compounds
Glucose-Asparagine: Another Amadori product formed from glucose and asparagine.
Fructose-Glutamine: Formed from fructose and glutamine, similar in structure and formation to fructose-asparagine.
Uniqueness
This compound is unique due to its specific utilization by Salmonella enterica as a primary nutrient. This specificity makes it a valuable target for studying bacterial metabolism and developing targeted antibacterial therapies .
Properties
IUPAC Name |
(2R)-4-amino-4-oxo-2-[[(3S,4R,5R)-2,3,4,5-tetrahydroxyoxan-2-yl]methylamino]butanoic acid | |
---|---|---|
Source | PubChem | |
URL | https://pubchem.ncbi.nlm.nih.gov | |
Description | Data deposited in or computed by PubChem | |
InChI |
InChI=1S/C10H18N2O8/c11-6(14)1-4(9(17)18)12-3-10(19)8(16)7(15)5(13)2-20-10/h4-5,7-8,12-13,15-16,19H,1-3H2,(H2,11,14)(H,17,18)/t4-,5-,7-,8+,10?/m1/s1 | |
Source | PubChem | |
URL | https://pubchem.ncbi.nlm.nih.gov | |
Description | Data deposited in or computed by PubChem | |
InChI Key |
AOBQWGHMIKNEHI-CWOUCCJVSA-N | |
Source | PubChem | |
URL | https://pubchem.ncbi.nlm.nih.gov | |
Description | Data deposited in or computed by PubChem | |
Canonical SMILES |
C1C(C(C(C(O1)(CNC(CC(=O)N)C(=O)O)O)O)O)O | |
Source | PubChem | |
URL | https://pubchem.ncbi.nlm.nih.gov | |
Description | Data deposited in or computed by PubChem | |
Isomeric SMILES |
C1[C@H]([C@H]([C@@H](C(O1)(CN[C@H](CC(=O)N)C(=O)O)O)O)O)O | |
Source | PubChem | |
URL | https://pubchem.ncbi.nlm.nih.gov | |
Description | Data deposited in or computed by PubChem | |
Molecular Formula |
C10H18N2O8 | |
Record name | Fructose-asparagine | |
Source | Wikipedia | |
URL | https://en.wikipedia.org/wiki/Fructose-asparagine | |
Description | Chemical information link to Wikipedia. | |
Source | PubChem | |
URL | https://pubchem.ncbi.nlm.nih.gov | |
Description | Data deposited in or computed by PubChem | |
DSSTOX Substance ID |
DTXSID70747834 | |
Record name | (2R)-4-Amino-4-oxo-2-({[(3S,4R,5R)-2,3,4,5-tetrahydroxyoxan-2-yl]methyl}amino)butanoic acid (non-preferred name) | |
Source | EPA DSSTox | |
URL | https://comptox.epa.gov/dashboard/DTXSID70747834 | |
Description | DSSTox provides a high quality public chemistry resource for supporting improved predictive toxicology. | |
Molecular Weight |
294.26 g/mol | |
Source | PubChem | |
URL | https://pubchem.ncbi.nlm.nih.gov | |
Description | Data deposited in or computed by PubChem | |
CAS No. |
34393-27-6 | |
Record name | (2R)-4-Amino-4-oxo-2-({[(3S,4R,5R)-2,3,4,5-tetrahydroxyoxan-2-yl]methyl}amino)butanoic acid (non-preferred name) | |
Source | EPA DSSTox | |
URL | https://comptox.epa.gov/dashboard/DTXSID70747834 | |
Description | DSSTox provides a high quality public chemistry resource for supporting improved predictive toxicology. | |
Disclaimer and Information on In-Vitro Research Products
Please be aware that all articles and product information presented on BenchChem are intended solely for informational purposes. The products available for purchase on BenchChem are specifically designed for in-vitro studies, which are conducted outside of living organisms. In-vitro studies, derived from the Latin term "in glass," involve experiments performed in controlled laboratory settings using cells or tissues. It is important to note that these products are not categorized as medicines or drugs, and they have not received approval from the FDA for the prevention, treatment, or cure of any medical condition, ailment, or disease. We must emphasize that any form of bodily introduction of these products into humans or animals is strictly prohibited by law. It is essential to adhere to these guidelines to ensure compliance with legal and ethical standards in research and experimentation.