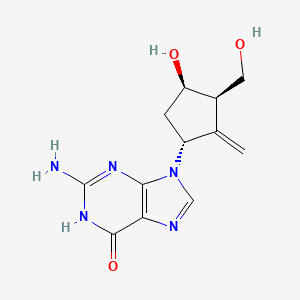
(1R,3R,4R)-Entecavir
- Click on QUICK INQUIRY to receive a quote from our team of experts.
- With the quality product at a COMPETITIVE price, you can focus more on your research.
Overview
Description
(1R,3R,4R)-Entecavir is a synthetic nucleoside analogue with potent antiviral properties. It is primarily used in the treatment of chronic hepatitis B virus infection. The compound works by inhibiting the reverse transcriptase enzyme, which is crucial for the replication of the hepatitis B virus. This inhibition helps to reduce the viral load in patients, thereby improving liver function and reducing the risk of liver damage.
Preparation Methods
Synthetic Routes and Reaction Conditions: The synthesis of (1R,3R,4R)-Entecavir involves multiple steps, starting from commercially available starting materials. The key steps include the formation of the cyclopentane ring, introduction of the hydroxyl groups, and the attachment of the guanine base. The reaction conditions typically involve the use of protecting groups to ensure selective reactions at specific sites on the molecule.
Industrial Production Methods: Industrial production of this compound follows a similar synthetic route but is optimized for large-scale production. This involves the use of high-yielding reactions, efficient purification methods, and stringent quality control measures to ensure the purity and efficacy of the final product.
Chemical Reactions Analysis
Types of Reactions: (1R,3R,4R)-Entecavir undergoes various chemical reactions, including:
Oxidation: The hydroxyl groups can be oxidized to form ketones or aldehydes.
Reduction: The compound can be reduced to form different derivatives with altered biological activity.
Substitution: The guanine base can undergo substitution reactions to introduce different functional groups.
Common Reagents and Conditions:
Oxidation: Reagents such as potassium permanganate or chromium trioxide are commonly used.
Reduction: Sodium borohydride or lithium aluminum hydride are typical reducing agents.
Substitution: Halogenating agents like thionyl chloride or phosphorus tribromide are used for substitution reactions.
Major Products: The major products formed from these reactions include various derivatives of this compound with modified antiviral properties. These derivatives are often studied for their potential use in treating other viral infections or for improving the pharmacokinetic properties of the original compound.
Scientific Research Applications
(1R,3R,4R)-Entecavir has a wide range of scientific research applications, including:
Chemistry: It is used as a model compound for studying nucleoside analogues and their chemical properties.
Biology: Researchers use this compound to study the mechanisms of viral replication and the development of antiviral resistance.
Medicine: The compound is extensively studied for its therapeutic potential in treating hepatitis B and other viral infections.
Industry: this compound is used in the pharmaceutical industry for the development of new antiviral drugs and formulations.
Mechanism of Action
The mechanism of action of (1R,3R,4R)-Entecavir involves the inhibition of the hepatitis B virus reverse transcriptase enzyme. This enzyme is responsible for converting viral RNA into DNA, a crucial step in the viral replication cycle. By inhibiting this enzyme, this compound prevents the virus from replicating, thereby reducing the viral load in the patient’s body. The compound specifically targets the active site of the reverse transcriptase enzyme, blocking its activity and leading to the termination of the viral DNA chain.
Comparison with Similar Compounds
(1R,3R,4R)-Entecavir is unique among nucleoside analogues due to its high potency and selectivity for the hepatitis B virus reverse transcriptase enzyme. Similar compounds include:
Lamivudine: Another nucleoside analogue used to treat hepatitis B, but with a higher risk of developing resistance.
Adefovir: A nucleotide analogue with a broader spectrum of activity but lower potency compared to this compound.
Tenofovir: A nucleotide analogue with high potency but associated with renal toxicity in some patients.
The uniqueness of this compound lies in its ability to achieve high levels of viral suppression with a low risk of resistance and minimal side effects, making it a preferred choice for long-term treatment of chronic hepatitis B.
Properties
IUPAC Name |
2-amino-9-[(1R,3R,4R)-4-hydroxy-3-(hydroxymethyl)-2-methylidenecyclopentyl]-1H-purin-6-one |
Source
|
---|---|---|
Source | PubChem | |
URL | https://pubchem.ncbi.nlm.nih.gov | |
Description | Data deposited in or computed by PubChem | |
InChI |
InChI=1S/C12H15N5O3/c1-5-6(3-18)8(19)2-7(5)17-4-14-9-10(17)15-12(13)16-11(9)20/h4,6-8,18-19H,1-3H2,(H3,13,15,16,20)/t6-,7+,8+/m0/s1 |
Source
|
Source | PubChem | |
URL | https://pubchem.ncbi.nlm.nih.gov | |
Description | Data deposited in or computed by PubChem | |
InChI Key |
QDGZDCVAUDNJFG-XLPZGREQSA-N |
Source
|
Source | PubChem | |
URL | https://pubchem.ncbi.nlm.nih.gov | |
Description | Data deposited in or computed by PubChem | |
Canonical SMILES |
C=C1C(CC(C1CO)O)N2C=NC3=C2N=C(NC3=O)N |
Source
|
Source | PubChem | |
URL | https://pubchem.ncbi.nlm.nih.gov | |
Description | Data deposited in or computed by PubChem | |
Isomeric SMILES |
C=C1[C@@H](C[C@H]([C@H]1CO)O)N2C=NC3=C2N=C(NC3=O)N |
Source
|
Source | PubChem | |
URL | https://pubchem.ncbi.nlm.nih.gov | |
Description | Data deposited in or computed by PubChem | |
Molecular Formula |
C12H15N5O3 |
Source
|
Source | PubChem | |
URL | https://pubchem.ncbi.nlm.nih.gov | |
Description | Data deposited in or computed by PubChem | |
Molecular Weight |
277.28 g/mol |
Source
|
Source | PubChem | |
URL | https://pubchem.ncbi.nlm.nih.gov | |
Description | Data deposited in or computed by PubChem | |
Disclaimer and Information on In-Vitro Research Products
Please be aware that all articles and product information presented on BenchChem are intended solely for informational purposes. The products available for purchase on BenchChem are specifically designed for in-vitro studies, which are conducted outside of living organisms. In-vitro studies, derived from the Latin term "in glass," involve experiments performed in controlled laboratory settings using cells or tissues. It is important to note that these products are not categorized as medicines or drugs, and they have not received approval from the FDA for the prevention, treatment, or cure of any medical condition, ailment, or disease. We must emphasize that any form of bodily introduction of these products into humans or animals is strictly prohibited by law. It is essential to adhere to these guidelines to ensure compliance with legal and ethical standards in research and experimentation.