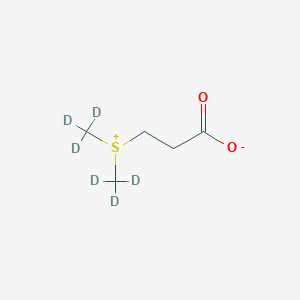
Dimethylsulfonioproprionate-d6
- Click on QUICK INQUIRY to receive a quote from our team of experts.
- With the quality product at a COMPETITIVE price, you can focus more on your research.
Overview
Description
Dimethylsulfonioproprionate-d6 (DMSP-d6) is an organosulfur compound with the molecular formula C5H4D6O2S and a molecular weight of 140.23 . It is a zwitterionic metabolite found in marine phytoplankton, seaweeds, and some species of terrestrial and aquatic vascular plants . It is known to play several physiological and environmental roles .
Synthesis Analysis
DMSP is biosynthesized from S-methylmethionine with two intermediates, dimethylsulfoniumpropylamine and dimethylsulfoniumpropionaldehyde . In algae, the biosynthesis starts with the replacement of the amino group in methionine by hydroxide . A key step in this pathway is catalyzed by the DsyB enzyme .Molecular Structure Analysis
The molecular structure of DMSP-d6 is represented by the formula C5H4D6O2S . The compound is a colourless to pale yellow oil .Chemical Reactions Analysis
DMSP is broken down by marine microbes to form two major volatile sulfur products, each with distinct effects on the environment. One of its breakdown products is methanethiol (CH3SH), which is assimilated by bacteria into protein sulfur . Another volatile breakdown product is dimethyl sulfide (CH3SCH3; DMS) .Physical And Chemical Properties Analysis
DMSP-d6 is a liquid that should be stored at 4° C . It appears as a clear pale yellow oil .Scientific Research Applications
Marine Microbial Communities
DMSP-d6 plays a crucial role in the metabolism of marine microbial communities. It serves as a key organic sulfur compound produced by phytoplankton and macrophytes, and is metabolized by heterotrophic bacterioplankton . The uptake and accumulation of DMSP-d6 by different fractions of the microbial community can significantly influence the microbial composition and abundance of DMSP degrading genes, affecting sulfur cycling in marine environments .
Climate Regulation
DMSP-d6 is involved in climate regulation through its conversion to dimethyl sulfide (DMS), a climatically active aerosol. The microbial communities’ response to DMSP-d6 enrichment varies, with some preferring to cleave DMSP into DMS, which has implications for atmospheric sulfur levels and potentially climate change .
Stress Protection in Marine Organisms
DMSP-d6 functions as a cryoprotectant and osmolyte, providing stress protection to marine organisms. It helps in maintaining cell integrity against varying salinity and temperature, which is vital for the survival of marine phytoplankton and bacteria .
Chemotaxis and Nutrient Cycling
DMSP-d6 serves as a chemoattractant for marine organisms, playing a role in chemotaxis. It also contributes to nutrient cycling by acting as a source of carbon and sulfur for marine microbes, thus supporting the marine food web .
Alternative Biosynthesis Pathways
Research has uncovered alternative biosynthesis enzymes for DMSP-d6 in diverse and abundant microbes. This discovery expands the understanding of DMSP production beyond traditional pathways and highlights the role of these enzymes in global sulfur cycling .
Sea Ice Microbial Activity
DMSP-d6 and its derivative, dimethylsulfoxide (DMSO), are produced in high concentrations by microbial communities in sea ice. These metabolites are involved in the cycling of the climate-cooling gas dimethylsulfide and play a role in the extreme environment of sea ice .
Sulfur Cycling in Oligotrophic Waters
In oligotrophic waters, such as those found in the Great Barrier Reef, DMSP-d6 uptake by microbial communities is a key factor in sulfur cycling. The utilization of DMSP-d6 by cells in these nutrient-limited environments provides insights into the metabolic processes of deeper water microbial communities .
Global DMSP Production
The presence of DMSP-d6 biosynthesis enzymes in diverse and environmentally abundant algae, particularly bloom-forming Pelagophyceae species, indicates a significant contribution to global DMSP production. These algae are now recognized as more abundant DMSP producers than previously known, emphasizing their importance in global sulfur cycling .
Mechanism of Action
Target of Action
Dimethylsulfonioproprionate (DMSP) is primarily targeted by marine organisms, including plants, marine algae, and dinoflagellates . It is also targeted by certain bacteria, which have been found to play a significant role in the global sulfur cycle .
Mode of Action
DMSP interacts with its targets through a process known as bacterial catabolism . In this process, DMSP is degraded by marine bacteria to produce dimethylsulfide (DMS), a climatically important gas . An alternative pathway results in the release of methanethiol, a highly reactive volatile sulfur compound .
Biochemical Pathways
DMSP is involved in several biochemical pathways. It is synthesized from methionine (Met) via three distinct synthesis pathways . The degradation of DMSP can occur via cleavage to DMS and acrylic acid or demethylation and demethiolation to methanethiol . These reactions are catalyzed by various enzymes, including DddX, which catalyzes a two-step reaction: the ligation of DMSP and CoA, and the cleavage of DMSP-CoA to produce DMS and acryloyl-CoA .
Pharmacokinetics
It is known that dmsp is a highly abundant sulfur metabolite in marine ecosystems , suggesting that it is readily absorbed and distributed in these environments.
Result of Action
The action of DMSP has significant molecular and cellular effects. It has been shown to promote longevity and prevent age-associated functional decline in Caenorhabditis elegans, a soil-dwelling organism . DMSP achieves this by decreasing mitochondrial content and improving mitochondrial function in C. elegans at the old stage, which is achieved via enhancing autophagy flux .
Action Environment
The action of DMSP is influenced by environmental factors. It is produced in massive quantities by phytoplankton in the North Atlantic and Antarctic oceans . The degradation of DMSP by marine bacteria ensures an important role for both compounds in the global sulfur cycle . Furthermore, bacteria have been found to be key DMSP producers in deep seawater and sediment , indicating that the action, efficacy, and stability of DMSP are influenced by the marine environment.
Future Directions
The study of DMSP and its derivatives continues to be a significant area of research due to their roles in global sulfur cycling and atmospheric chemistry . Future studies may focus on the discovery and characterization of novel substrates and enzymes involved in organosulfur cycling, as well as investigations of deep sea and sedimentary organic sulfur .
properties
IUPAC Name |
3-[bis(trideuteriomethyl)sulfonio]propanoate |
Source
|
---|---|---|
Source | PubChem | |
URL | https://pubchem.ncbi.nlm.nih.gov | |
Description | Data deposited in or computed by PubChem | |
InChI |
InChI=1S/C5H10O2S/c1-8(2)4-3-5(6)7/h3-4H2,1-2H3/i1D3,2D3 |
Source
|
Source | PubChem | |
URL | https://pubchem.ncbi.nlm.nih.gov | |
Description | Data deposited in or computed by PubChem | |
InChI Key |
DFPOZTRSOAQFIK-WFGJKAKNSA-N |
Source
|
Source | PubChem | |
URL | https://pubchem.ncbi.nlm.nih.gov | |
Description | Data deposited in or computed by PubChem | |
Canonical SMILES |
C[S+](C)CCC(=O)[O-] |
Source
|
Source | PubChem | |
URL | https://pubchem.ncbi.nlm.nih.gov | |
Description | Data deposited in or computed by PubChem | |
Isomeric SMILES |
[2H]C([2H])([2H])[S+](CCC(=O)[O-])C([2H])([2H])[2H] |
Source
|
Source | PubChem | |
URL | https://pubchem.ncbi.nlm.nih.gov | |
Description | Data deposited in or computed by PubChem | |
Molecular Formula |
C5H10O2S |
Source
|
Source | PubChem | |
URL | https://pubchem.ncbi.nlm.nih.gov | |
Description | Data deposited in or computed by PubChem | |
Molecular Weight |
140.24 g/mol |
Source
|
Source | PubChem | |
URL | https://pubchem.ncbi.nlm.nih.gov | |
Description | Data deposited in or computed by PubChem | |
Disclaimer and Information on In-Vitro Research Products
Please be aware that all articles and product information presented on BenchChem are intended solely for informational purposes. The products available for purchase on BenchChem are specifically designed for in-vitro studies, which are conducted outside of living organisms. In-vitro studies, derived from the Latin term "in glass," involve experiments performed in controlled laboratory settings using cells or tissues. It is important to note that these products are not categorized as medicines or drugs, and they have not received approval from the FDA for the prevention, treatment, or cure of any medical condition, ailment, or disease. We must emphasize that any form of bodily introduction of these products into humans or animals is strictly prohibited by law. It is essential to adhere to these guidelines to ensure compliance with legal and ethical standards in research and experimentation.