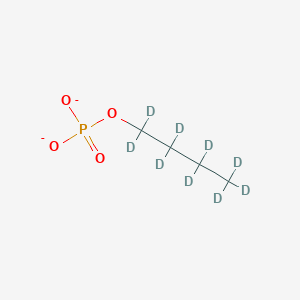
Monobutyl Phosphate-d9
- Click on QUICK INQUIRY to receive a quote from our team of experts.
- With the quality product at a COMPETITIVE price, you can focus more on your research.
Overview
Description
Monobutyl Phosphate-d9 is a deuterated compound with the molecular formula C4H2D9O4P and a molecular weight of 163.16. It is a labeled analogue of Monobutyl Phosphate, where the hydrogen atoms are replaced with deuterium. This compound is primarily used in research settings, particularly in nuclear magnetic resonance (NMR) spectroscopy due to its unique isotopic labeling .
Preparation Methods
Monobutyl Phosphate-d9 is typically synthesized through the reaction of deuterated acids or their sodium salts with the corresponding alcohols to form the desired deuterated esters. The process involves the following steps:
Synthesis of Deuterated Alcohol: The deuterated alcohol is prepared by reacting deuterium oxide (D2O) with the corresponding alcohol.
Esterification: The deuterated alcohol is then reacted with phosphoric acid or its salts under controlled conditions to produce this compound.
Industrial production methods for this compound are similar to laboratory synthesis but are scaled up to meet the demand for research and industrial applications.
Chemical Reactions Analysis
Monobutyl Phosphate-d9 undergoes various chemical reactions, including:
Oxidation: It can be oxidized to form corresponding phosphates.
Reduction: Reduction reactions can convert it back to the corresponding alcohol and phosphoric acid.
Substitution: It can undergo nucleophilic substitution reactions where the butyl group is replaced by other nucleophiles.
Common reagents used in these reactions include oxidizing agents like potassium permanganate, reducing agents like lithium aluminum hydride, and nucleophiles like sodium hydroxide. The major products formed depend on the specific reaction conditions and reagents used .
Scientific Research Applications
Monobutyl Phosphate-d9 has a wide range of applications in scientific research:
Chemistry: Used as a standard in NMR spectroscopy to study molecular structures and dynamics.
Biology: Employed in metabolic studies to trace biochemical pathways.
Medicine: Utilized in pharmacokinetic studies to understand drug metabolism and distribution.
Industry: Acts as a surfactant in various industrial processes due to its stability in alkaline conditions
Mechanism of Action
The mechanism of action of Monobutyl Phosphate-d9 involves its interaction with molecular targets through its phosphate group. The deuterium atoms provide a unique isotopic signature that can be detected using NMR spectroscopy, allowing researchers to study the compound’s behavior in various environments. The phosphate group can participate in hydrogen bonding and electrostatic interactions, influencing the compound’s reactivity and stability .
Comparison with Similar Compounds
Monobutyl Phosphate-d9 is unique due to its deuterium labeling, which distinguishes it from other similar compounds such as:
Monobutyl Phosphate: The non-deuterated analogue.
Dibutyl Phosphate: Contains two butyl groups instead of one.
Tributyl Phosphate: Contains three butyl groups and is commonly used as a solvent and plasticizer.
The deuterium labeling in this compound provides enhanced stability and distinct NMR signals, making it particularly valuable in research applications .
Properties
IUPAC Name |
1,1,2,2,3,3,4,4,4-nonadeuteriobutyl phosphate |
Source
|
---|---|---|
Source | PubChem | |
URL | https://pubchem.ncbi.nlm.nih.gov | |
Description | Data deposited in or computed by PubChem | |
InChI |
InChI=1S/C4H11O4P/c1-2-3-4-8-9(5,6)7/h2-4H2,1H3,(H2,5,6,7)/p-2/i1D3,2D2,3D2,4D2 |
Source
|
Source | PubChem | |
URL | https://pubchem.ncbi.nlm.nih.gov | |
Description | Data deposited in or computed by PubChem | |
InChI Key |
BNMJSBUIDQYHIN-YNSOAAEFSA-L |
Source
|
Source | PubChem | |
URL | https://pubchem.ncbi.nlm.nih.gov | |
Description | Data deposited in or computed by PubChem | |
Canonical SMILES |
CCCCOP(=O)([O-])[O-] |
Source
|
Source | PubChem | |
URL | https://pubchem.ncbi.nlm.nih.gov | |
Description | Data deposited in or computed by PubChem | |
Isomeric SMILES |
[2H]C([2H])([2H])C([2H])([2H])C([2H])([2H])C([2H])([2H])OP(=O)([O-])[O-] |
Source
|
Source | PubChem | |
URL | https://pubchem.ncbi.nlm.nih.gov | |
Description | Data deposited in or computed by PubChem | |
Molecular Formula |
C4H9O4P-2 |
Source
|
Source | PubChem | |
URL | https://pubchem.ncbi.nlm.nih.gov | |
Description | Data deposited in or computed by PubChem | |
Molecular Weight |
161.14 g/mol |
Source
|
Source | PubChem | |
URL | https://pubchem.ncbi.nlm.nih.gov | |
Description | Data deposited in or computed by PubChem | |
Disclaimer and Information on In-Vitro Research Products
Please be aware that all articles and product information presented on BenchChem are intended solely for informational purposes. The products available for purchase on BenchChem are specifically designed for in-vitro studies, which are conducted outside of living organisms. In-vitro studies, derived from the Latin term "in glass," involve experiments performed in controlled laboratory settings using cells or tissues. It is important to note that these products are not categorized as medicines or drugs, and they have not received approval from the FDA for the prevention, treatment, or cure of any medical condition, ailment, or disease. We must emphasize that any form of bodily introduction of these products into humans or animals is strictly prohibited by law. It is essential to adhere to these guidelines to ensure compliance with legal and ethical standards in research and experimentation.