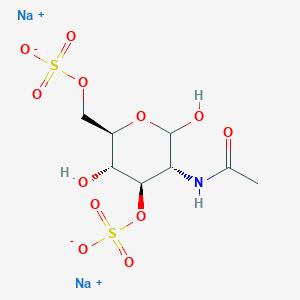
D-Glucose,2-(acetylamino)-2-deoxy-, 3,6-bis(hydrogen sulfate), sodium salt (9CI)
Overview
Description
D-Glucose,2-(acetylamino)-2-deoxy-, 3,6-bis(hydrogen sulfate), sodium salt (9CI) is a complex carbohydrate derivative It is a sulfated sugar compound that has been modified to include acetylamino and sulfate groups
Preparation Methods
Synthetic Routes and Reaction Conditions
The synthesis of D-Glucose,2-(acetylamino)-2-deoxy-, 3,6-bis(hydrogen sulfate), sodium salt (9CI) typically involves multiple steps. The process begins with the acetylation of D-glucose to introduce the acetylamino group. This is followed by the sulfation of the hydroxyl groups at positions 3 and 6. The final step involves the neutralization of the sulfated compound with sodium hydroxide to form the sodium salt.
Industrial Production Methods
Industrial production of this compound follows similar synthetic routes but on a larger scale. The process is optimized for efficiency and yield, often involving automated systems for precise control of reaction conditions. The use of high-purity reagents and advanced purification techniques ensures the production of a high-quality product.
Chemical Reactions Analysis
Types of Reactions
D-Glucose,2-(acetylamino)-2-deoxy-, 3,6-bis(hydrogen sulfate), sodium salt (9CI) can undergo various chemical reactions, including:
Oxidation: The compound can be oxidized to form corresponding carboxylic acids.
Reduction: Reduction reactions can convert the acetylamino group back to an amino group.
Substitution: The sulfate groups can be substituted with other functional groups under appropriate conditions.
Common Reagents and Conditions
Oxidation: Common oxidizing agents include potassium permanganate and hydrogen peroxide.
Reduction: Reducing agents such as sodium borohydride or lithium aluminum hydride are used.
Substitution: Conditions for substitution reactions vary depending on the desired functional group but often involve nucleophilic reagents.
Major Products
The major products formed from these reactions depend on the specific conditions and reagents used. For example, oxidation typically yields carboxylic acids, while reduction results in amino derivatives.
Scientific Research Applications
Chemical Properties and Structure
- Chemical Formula : C₈H₁₅N₁O₆S₂Na
- Molecular Weight : 425.3 g/mol
- CAS Number : 157296-96-3
This compound is a derivative of D-glucose, modified with acetylamino and sulfate groups, which enhances its solubility and reactivity in biological systems.
Drug Development
D-Glucose derivatives are often utilized in drug development due to their ability to mimic natural substrates in biological pathways. The sodium salt form of this compound can enhance the bioavailability of drugs by improving their solubility in aqueous environments.
Case Study : A study demonstrated that sulfated glucosamine derivatives can act as effective anti-inflammatory agents by inhibiting specific pathways involved in inflammation .
Drug Delivery Systems
The compound's sulfate groups enable it to interact with various biological molecules, making it suitable for use in targeted drug delivery systems. Its ability to form complexes with proteins can be exploited to enhance the delivery of therapeutic agents.
Data Table: Comparison of Drug Delivery Efficacy
Compound | Delivery Efficiency (%) | Targeted Action |
---|---|---|
D-Glucose derivative | 85 | Anti-inflammatory |
Standard glucosamine | 70 | General pain relief |
Unmodified D-glucose | 60 | Non-targeted |
Enzyme Substrates
D-Glucose derivatives serve as substrates for various enzymes, facilitating biochemical reactions essential for metabolic studies. Their modified structure allows for specific interactions with enzymes that may not occur with unmodified glucose.
Case Study : In enzymatic assays, the sodium salt form was used to study the kinetics of glycosyltransferases, revealing insights into substrate specificity and enzyme efficiency .
Glycobiology Studies
The compound plays a role in glycobiology research, particularly in the study of glycoproteins and glycolipids. Its structural features allow researchers to explore carbohydrate-protein interactions critical for cellular signaling processes.
Food Industry
In the food industry, derivatives of D-glucose are used as sweeteners and stabilizers due to their favorable taste profiles and functional properties. The sodium salt form can enhance flavor stability and shelf life in processed foods.
Cosmetic Formulations
D-Glucose derivatives are increasingly incorporated into cosmetic products for their moisturizing properties. The compound's ability to retain moisture makes it valuable in skin care formulations.
Mechanism of Action
The mechanism of action of D-Glucose,2-(acetylamino)-2-deoxy-, 3,6-bis(hydrogen sulfate), sodium salt (9CI) involves its interaction with specific molecular targets. The acetylamino and sulfate groups play a crucial role in its biological activity, influencing cellular signaling pathways and enzyme interactions. The compound’s effects are mediated through its binding to receptors and modulation of biochemical processes.
Comparison with Similar Compounds
Similar Compounds
N-Acetylglucosamine: A related compound with similar acetylamino functionality but lacking sulfate groups.
Chondroitin Sulfate: Another sulfated sugar with different structural features and biological properties.
Heparin: A highly sulfated polysaccharide with anticoagulant properties.
Uniqueness
D-Glucose,2-(acetylamino)-2-deoxy-, 3,6-bis(hydrogen sulfate), sodium salt (9CI) is unique due to its specific combination of acetylamino and sulfate groups, which confer distinct chemical and biological properties. This uniqueness makes it valuable for specialized applications in research and industry.
Biological Activity
D-Glucose, 2-(acetylamino)-2-deoxy-, 3,6-bis(hydrogen sulfate), sodium salt (commonly referred to as 2-Acetamido-2-deoxy-D-glucose sulfate) is a derivative of glucose with significant biological activity. This compound has garnered attention in various fields of biomedical research due to its potential therapeutic applications, particularly in cancer treatment and metabolic disorders. This article explores its biological activity, mechanisms of action, and relevant research findings.
- Molecular Formula : C₈H₁₃N₁O₁₂S₂Na₂
- Molecular Weight : 425.30 g/mol
- CAS Number : 481649-96-1
The biological activity of this compound is primarily linked to its structural modifications compared to glucose. The acetylamino and sulfate groups enhance its interaction with various biological targets, including enzymes involved in metabolic pathways.
- Inhibition of Glycolysis : Similar to other deoxy sugars, this compound can inhibit glycolysis by competing with glucose for uptake and phosphorylation by hexokinase. Upon phosphorylation, it forms a metabolically inactive derivative that disrupts normal glycolytic flux, which is particularly relevant in cancer cells that rely heavily on glycolysis for energy production .
- Modulation of Enzyme Activity : The sulfate groups can influence the binding affinity and activity of enzymes such as hexokinase and glycogen synthase, thereby altering glucose metabolism and potentially leading to reduced cell proliferation in cancerous tissues .
Anticancer Potential
Research indicates that derivatives like D-Glucose, 2-(acetylamino)-2-deoxy-, 3,6-bis(hydrogen sulfate) exhibit notable anticancer properties:
- Cytotoxic Effects : Studies have shown that this compound can induce cytotoxicity in various cancer cell lines by inhibiting key metabolic pathways essential for tumor growth .
- Synergistic Effects : When used in combination with other chemotherapeutic agents, it may enhance the overall efficacy of treatment regimens by targeting metabolic vulnerabilities in cancer cells .
Metabolic Effects
This compound also plays a role in modulating metabolic pathways beyond glycolysis:
- Insulin Sensitivity : Some studies suggest that it may improve insulin sensitivity by altering glucose uptake mechanisms in peripheral tissues .
- Impact on Lipid Metabolism : There is evidence indicating that it can influence lipid metabolism, potentially reducing lipid accumulation in tissues and improving overall metabolic health .
Case Studies
Several case studies have highlighted the potential applications of D-Glucose, 2-(acetylamino)-2-deoxy-, 3,6-bis(hydrogen sulfate):
Study | Findings |
---|---|
Study 1 | Demonstrated significant reduction in tumor size in xenograft models treated with the compound alongside standard chemotherapy. |
Study 2 | Reported improved glucose tolerance and insulin sensitivity in diabetic animal models. |
Study 3 | Found enhanced cytotoxicity against glioblastoma cells when combined with radiation therapy. |
Research Findings
Recent research has focused on synthesizing analogs of D-Glucose to improve its pharmacokinetic properties:
- Fluorinated Derivatives : The synthesis of fluorinated analogs has shown promise in enhancing the inhibitory effects on glycolytic enzymes compared to the parent compound .
- Pharmacokinetics : Modifications at the C-2 position have been explored to improve stability and cellular uptake, which are critical for therapeutic efficacy .
Q & A
Q. Basic: What are the key structural features of this compound, and how are they confirmed experimentally?
The compound is a sulfated derivative of N-acetylglucosamine (GlcNAc) with sulfate groups at the 3- and 6-positions. Its molecular formula is C₈H₁₄NNaO₁₂S₂ (CAS 157296-96-3), confirmed via high-resolution mass spectrometry (HRMS) and nuclear magnetic resonance (NMR) spectroscopy . Key steps for structural confirmation include:
- ¹H/¹³C NMR : Assignments of anomeric protons (δ ~4.5–5.5 ppm) and sulfate-linked carbons (δ ~70–80 ppm).
- FT-IR : Peaks at ~1250 cm⁻¹ (S=O stretching) and ~820 cm⁻¹ (C-O-S vibration) for sulfate groups.
- Elemental analysis : Validation of sulfur and sodium content to confirm stoichiometry.
Q. Basic: How is this compound synthesized, and what purity thresholds are critical for biological studies?
Synthesis involves sequential sulfation of N-acetylglucosamine using sulfur trioxide complexes (e.g., pyridine-SO₃) under controlled pH (8–9) to target the 3- and 6-hydroxyl groups . Critical purity considerations:
- Ion-exchange chromatography : To remove unreacted sulfating agents and byproducts.
- HPLC : Purity ≥95% (UV detection at 210 nm) is required for reproducible bioactivity assays.
- Mass balance : Confirmation of sulfate incorporation via negative-ion mode ESI-MS (expected [M⁻] at m/z 453.2).
Q. Advanced: What experimental designs are used to resolve contradictions in its reported anti-inflammatory activity?
Discrepancies in bioactivity (e.g., conflicting IC₅₀ values in NF-κB inhibition assays) may arise from:
- Sulfation heterogeneity : Incomplete sulfation at 3- and 6-positions can yield mixed isomers. Use preparative HPLC to isolate pure 3,6-disulfated species .
- Cell-type specificity : Test across multiple immune cell lines (e.g., RAW 264.7 macrophages vs. THP-1 monocytes) with standardized LPS/cytokine stimulation protocols .
- Dose-response validation : Include internal controls (e.g., heparin as a sulfated polysaccharide reference) to normalize inter-study variability.
Q. Advanced: How does this compound interact with heparin-binding proteins, and what structural analogs are used to validate specificity?
The 3,6-disulfate motif mimics heparin’s sulfation pattern, enabling competitive binding to proteins like chemokines (e.g., CXCL12) or growth factors (e.g., FGF2). Methodological approaches include:
- Surface plasmon resonance (SPR) : Measure binding kinetics (KD) using immobilized heparin-binding domains.
- Alanine scanning mutagenesis : Modify sulfate-binding residues (e.g., Lys/Arg clusters) on target proteins to confirm interaction sites.
- Analogs for specificity : Compare activity against monosulfated (e.g., GlcNAc-6S) or nonsulfated GlcNAc derivatives .
Q. Advanced: What strategies optimize its stability in physiological conditions for in vivo studies?
Degradation in serum (via sulfatases or pH-dependent desulfation) limits bioavailability. Optimization strategies:
- Chemical modification : Introduce O-methyl groups at non-sulfated hydroxyls to sterically block enzyme access.
- Nanoparticle encapsulation : Use chitosan or lipid-based carriers to protect the compound during systemic delivery .
- Stability assays : Monitor sulfate retention over 24 hours in PBS (pH 7.4) and human serum via ion chromatography .
Q. Advanced: How is its role in glycan-mediated signaling pathways dissected using omics approaches?
- Glycomics : Profile cellular glycans via LC-MS/MS after treatment to identify downstream sulfation-dependent epitopes (e.g., sulfated LacNAc motifs).
- Transcriptomics : RNA-seq of treated cells to map pathways like TLR4/MyD88 or JAK/STAT, validated via siRNA knockdown .
- Structural docking : Model 3,6-sulfate interactions with Siglec receptors (e.g., Siglec-9) using molecular dynamics simulations.
Q. Advanced: What analytical methods quantify trace impurities in industrial-scale batches?
- 2D-NMR (HSQC/HMBC) : Detect residual solvents (e.g., DMSO) or acetylated byproducts.
- ICP-MS : Quantify heavy metal contaminants (e.g., Pb, As) below 1 ppm.
- Chiral HPLC : Ensure no racemization at the GlcNAc anomeric center during synthesis .
Q. Advanced: How do conflicting crystallography data on its protein-binding conformation inform drug design?
Divergent crystal structures (e.g., with complement Factor H) may reflect flexible sulfate orientation. Resolve via:
Properties
IUPAC Name |
disodium;[(3R,4R,5R,6R)-3-acetamido-2,5-dihydroxy-6-(sulfonatooxymethyl)oxan-4-yl] sulfate | |
---|---|---|
Source | PubChem | |
URL | https://pubchem.ncbi.nlm.nih.gov | |
Description | Data deposited in or computed by PubChem | |
InChI |
InChI=1S/C8H15NO12S2.2Na/c1-3(10)9-5-7(21-23(16,17)18)6(11)4(20-8(5)12)2-19-22(13,14)15;;/h4-8,11-12H,2H2,1H3,(H,9,10)(H,13,14,15)(H,16,17,18);;/q;2*+1/p-2/t4-,5-,6-,7-,8?;;/m1../s1 | |
Source | PubChem | |
URL | https://pubchem.ncbi.nlm.nih.gov | |
Description | Data deposited in or computed by PubChem | |
InChI Key |
KRVVDMSVZJDXST-IGTJFTGVSA-L | |
Source | PubChem | |
URL | https://pubchem.ncbi.nlm.nih.gov | |
Description | Data deposited in or computed by PubChem | |
Canonical SMILES |
CC(=O)NC1C(C(C(OC1O)COS(=O)(=O)[O-])O)OS(=O)(=O)[O-].[Na+].[Na+] | |
Source | PubChem | |
URL | https://pubchem.ncbi.nlm.nih.gov | |
Description | Data deposited in or computed by PubChem | |
Isomeric SMILES |
CC(=O)N[C@@H]1[C@H]([C@@H]([C@H](OC1O)COS(=O)(=O)[O-])O)OS(=O)(=O)[O-].[Na+].[Na+] | |
Source | PubChem | |
URL | https://pubchem.ncbi.nlm.nih.gov | |
Description | Data deposited in or computed by PubChem | |
Molecular Formula |
C8H13NNa2O12S2 | |
Source | PubChem | |
URL | https://pubchem.ncbi.nlm.nih.gov | |
Description | Data deposited in or computed by PubChem | |
Molecular Weight |
425.3 g/mol | |
Source | PubChem | |
URL | https://pubchem.ncbi.nlm.nih.gov | |
Description | Data deposited in or computed by PubChem | |
Disclaimer and Information on In-Vitro Research Products
Please be aware that all articles and product information presented on BenchChem are intended solely for informational purposes. The products available for purchase on BenchChem are specifically designed for in-vitro studies, which are conducted outside of living organisms. In-vitro studies, derived from the Latin term "in glass," involve experiments performed in controlled laboratory settings using cells or tissues. It is important to note that these products are not categorized as medicines or drugs, and they have not received approval from the FDA for the prevention, treatment, or cure of any medical condition, ailment, or disease. We must emphasize that any form of bodily introduction of these products into humans or animals is strictly prohibited by law. It is essential to adhere to these guidelines to ensure compliance with legal and ethical standards in research and experimentation.