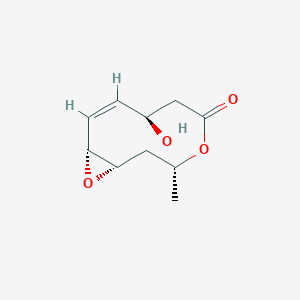
Decarestrictine A
Overview
Description
Decarestrictine A1 is a fungal metabolite discovered in Penicillium species . Its chemical structure is represented as follows:
Decarestrictine A1(CAS No.:127393−90−2)Molecular Formula: C10H14O4Molecular Weight: 198.22 g/mol
Mechanism of Action
Target of Action
Decarestrictine A is a member of the decarestrictines, a class of secondary metabolites isolated from various Penicillium strains It is known that decarestrictines exhibit interesting activity in cell line tests with hep-g2 liver cells due to an inhibitory effect on cholesterol biosynthesis .
Mode of Action
It is known that decarestrictines, including this compound, inhibit the de novo formation of cholesterol
Biochemical Pathways
this compound affects the biochemical pathway of cholesterol biosynthesis . By inhibiting this pathway, this compound can potentially disrupt the production of cholesterol, a crucial component of cell membranes and a precursor for the synthesis of other important biomolecules. The downstream effects of this disruption would depend on the specific cellular context and could include alterations in membrane fluidity and function, as well as changes in the synthesis of molecules derived from cholesterol.
Result of Action
The molecular and cellular effects of this compound’s action are likely to be diverse, given its role in inhibiting cholesterol biosynthesis . This could potentially affect a wide range of cellular processes, from membrane function to signal transduction.
Biochemical Analysis
Biochemical Properties
Decarestrictine A plays a crucial role in biochemical reactions, particularly in the inhibition of cholesterol biosynthesis. It interacts with several enzymes and proteins involved in this pathway. One of the primary targets of this compound is the enzyme 3-hydroxy-3-methylglutaryl-coenzyme A reductase, which is a key enzyme in the mevalonate pathway responsible for cholesterol synthesis. By inhibiting this enzyme, this compound effectively reduces the production of cholesterol. Additionally, this compound has been shown to interact with other biomolecules such as sterol regulatory element-binding proteins, which are transcription factors that regulate the expression of genes involved in lipid metabolism .
Cellular Effects
This compound exerts significant effects on various types of cells and cellular processes. In liver cells, this compound has been observed to inhibit cholesterol biosynthesis, leading to a reduction in intracellular cholesterol levels. This inhibition impacts cell signaling pathways, particularly those involving sterol regulatory element-binding proteins, which in turn affects gene expression related to lipid metabolism. Furthermore, this compound influences cellular metabolism by altering the balance of lipid synthesis and degradation, ultimately affecting overall cellular function .
Molecular Mechanism
The molecular mechanism of action of this compound involves its binding interactions with key enzymes and regulatory proteins in the cholesterol biosynthesis pathway. This compound binds to the active site of 3-hydroxy-3-methylglutaryl-coenzyme A reductase, inhibiting its activity and preventing the conversion of 3-hydroxy-3-methylglutaryl-coenzyme A to mevalonate. This inhibition leads to a decrease in the downstream production of cholesterol. Additionally, this compound modulates the activity of sterol regulatory element-binding proteins, affecting their ability to regulate gene expression related to lipid metabolism .
Temporal Effects in Laboratory Settings
In laboratory settings, the effects of this compound have been observed to change over time. Studies have shown that this compound is relatively stable under standard laboratory conditions, with minimal degradation over extended periods. Long-term exposure to this compound can lead to adaptive responses in cells, such as upregulation of alternative pathways for cholesterol synthesis or increased expression of efflux transporters to remove excess cholesterol. These adaptive responses can mitigate the inhibitory effects of this compound on cholesterol biosynthesis over time .
Dosage Effects in Animal Models
The effects of this compound vary with different dosages in animal models. At low doses, this compound effectively inhibits cholesterol biosynthesis without causing significant adverse effects. At higher doses, this compound can lead to toxic effects, including liver damage and disruption of lipid homeostasis. Threshold effects have been observed, where a certain dosage level is required to achieve significant inhibition of cholesterol biosynthesis. Beyond this threshold, increasing the dosage does not proportionally enhance the inhibitory effects but instead increases the risk of toxicity .
Metabolic Pathways
This compound is involved in the mevalonate pathway, a crucial metabolic pathway for the synthesis of cholesterol and other isoprenoids. By inhibiting 3-hydroxy-3-methylglutaryl-coenzyme A reductase, this compound disrupts the conversion of 3-hydroxy-3-methylglutaryl-coenzyme A to mevalonate, leading to a decrease in the production of downstream metabolites such as cholesterol, dolichol, and ubiquinone. This disruption affects the overall metabolic flux and levels of these metabolites, impacting various cellular processes .
Transport and Distribution
Within cells and tissues, this compound is transported and distributed through interactions with specific transporters and binding proteins. It has been observed to accumulate in the endoplasmic reticulum, where it exerts its inhibitory effects on cholesterol biosynthesis. Additionally, this compound can be transported to other cellular compartments, such as the Golgi apparatus and mitochondria, where it may influence other aspects of lipid metabolism. The distribution of this compound within cells is influenced by its interactions with lipid-binding proteins and transporters .
Subcellular Localization
This compound exhibits specific subcellular localization patterns that are crucial for its activity and function. It predominantly localizes to the endoplasmic reticulum, where it interacts with 3-hydroxy-3-methylglutaryl-coenzyme A reductase and other enzymes involved in cholesterol biosynthesis. Additionally, this compound may undergo post-translational modifications that direct it to specific compartments or organelles, enhancing its inhibitory effects on cholesterol biosynthesis. The subcellular localization of this compound is essential for its ability to modulate lipid metabolism and cellular function .
Preparation Methods
Industrial Production: Information on industrial-scale production methods for Decarestrictine A1 is limited. It is primarily studied in research settings rather than being produced on an industrial scale.
Chemical Reactions Analysis
Decarestrictine A1 may undergo various chemical reactions. While detailed studies are scarce, we can infer potential reactions based on its functional groups and structural features:
Oxidation and Reduction Reactions: Decarestrictine A1 likely participates in redox reactions due to its oxygen-containing functional groups.
Substitution Reactions: It may undergo nucleophilic or electrophilic substitutions.
Common Reagents and Conditions: Specific reagents and conditions remain undisclosed, but standard organic chemistry reagents (e.g., oxidants, reducing agents, Lewis acids) would likely play a role.
Major Products: The major products resulting from these reactions are not explicitly documented.
Scientific Research Applications
Decarestrictine A1’s applications span several scientific fields:
Chemistry: Researchers study its synthesis, reactivity, and potential as a building block for other compounds.
Biology: Investigations may focus on its biological activity, interactions with enzymes, and potential as a natural product.
Medicine: Decarestrictine A1’s pharmacological properties and potential therapeutic applications are areas of interest.
Industry: While industrial applications are limited, further research may uncover novel uses.
Properties
IUPAC Name |
(1S,3R,7R,8Z,10R)-7-hydroxy-3-methyl-4,11-dioxabicyclo[8.1.0]undec-8-en-5-one | |
---|---|---|
Source | PubChem | |
URL | https://pubchem.ncbi.nlm.nih.gov | |
Description | Data deposited in or computed by PubChem | |
InChI |
InChI=1S/C10H14O4/c1-6-4-9-8(14-9)3-2-7(11)5-10(12)13-6/h2-3,6-9,11H,4-5H2,1H3/b3-2-/t6-,7+,8-,9+/m1/s1 | |
Source | PubChem | |
URL | https://pubchem.ncbi.nlm.nih.gov | |
Description | Data deposited in or computed by PubChem | |
InChI Key |
JCWPVPJYCLLPQL-PHKLUEOISA-N | |
Source | PubChem | |
URL | https://pubchem.ncbi.nlm.nih.gov | |
Description | Data deposited in or computed by PubChem | |
Canonical SMILES |
CC1CC2C(O2)C=CC(CC(=O)O1)O | |
Source | PubChem | |
URL | https://pubchem.ncbi.nlm.nih.gov | |
Description | Data deposited in or computed by PubChem | |
Isomeric SMILES |
C[C@@H]1C[C@H]2[C@H](O2)/C=C\[C@@H](CC(=O)O1)O | |
Source | PubChem | |
URL | https://pubchem.ncbi.nlm.nih.gov | |
Description | Data deposited in or computed by PubChem | |
Molecular Formula |
C10H14O4 | |
Source | PubChem | |
URL | https://pubchem.ncbi.nlm.nih.gov | |
Description | Data deposited in or computed by PubChem | |
Molecular Weight |
198.22 g/mol | |
Source | PubChem | |
URL | https://pubchem.ncbi.nlm.nih.gov | |
Description | Data deposited in or computed by PubChem | |
A: Decarestrictine A is a 10-membered lactone, a type of organic compound, that belongs to the decarestrictine family. It is primarily known for its ability to inhibit cholesterol biosynthesis. [, , , ]
A: this compound is produced by certain fungal species, notably Penicillium simplicissimum and Penicillium corylophilum. [, ]
A: Yes, in vitro studies using the HEP-G2 cell line have confirmed the cholesterol biosynthesis inhibition by this compound. This effect has also been observed in vivo, further supporting its potential as a cholesterol-lowering agent. []
ANone: Unfortunately, the provided abstracts do not explicitly state the molecular formula and weight of this compound. Further research in chemical databases or the original research papers would be necessary to obtain this information.
A: this compound shares a common structural motif with other decarestrictines: a 10-membered lactone ring. The specific structural differences between this compound and other members of the family, such as variations in the oxygenation pattern between C-3 and C-7, are not detailed in these abstracts. []
A: While the provided abstracts don't offer specific spectroscopic data for this compound, they mention that its structure, along with other decarestrictines, has been elucidated using various spectroscopic techniques. These techniques likely include Nuclear Magnetic Resonance (NMR) spectroscopy and High-Resolution Electrospray Ionization Mass Spectrometry (HR-ESI-MS). [, , ]
A: Decarestrictines are produced through a polyketide biosynthetic pathway. Research using isotope-labeled precursors, like 13C-labeled acetates and malonic acid, has confirmed their origin from a common pentaketide precursor. []
A: Yes, manipulating the pH during fermentation can significantly influence the production of specific decarestrictines. This manipulation allows researchers to direct the fermentation process to yield desired members of the decarestrictine family. [, ]
A: Yes, this compound belongs to a family of structurally related compounds called decarestrictines. These compounds share a 10-membered lactone ring but differ in their oxygenation patterns and substituents. Examples of other decarestrictines include Decarestrictine B, C1, C2, D, E, F, G, H, I, J, L, M, N, and O. [, , , , , , , , , , , , , , , , , , , ]
A: this compound and D are members of the same family of compounds and share a similar core structure. During fermentation, this compound can be converted into Decarestrictine D under acidic conditions. This conversion is not enzyme-catalyzed, but rather a non-enzymatic process, highlighting the complex interplay of factors influencing the production of specific decarestrictines. []
A: Yes, several decarestrictines, including Decarestrictine D, I, J, L, and O, have been successfully synthesized. These syntheses typically involve multiple steps and often employ strategies like ring-closing metathesis (RCM) and stereoselective reactions to construct the 10-membered lactone ring and install the correct stereochemistry. [, , , , , , , , , , , , , ]
A: Yes, recent research has identified a polyketide cyclase enzyme, DcsB, involved in the biosynthesis of Decarestrictine C1. DcsB catalyzes the formation of the medium-ring lactone structure, a challenging step in chemical synthesis. []
Disclaimer and Information on In-Vitro Research Products
Please be aware that all articles and product information presented on BenchChem are intended solely for informational purposes. The products available for purchase on BenchChem are specifically designed for in-vitro studies, which are conducted outside of living organisms. In-vitro studies, derived from the Latin term "in glass," involve experiments performed in controlled laboratory settings using cells or tissues. It is important to note that these products are not categorized as medicines or drugs, and they have not received approval from the FDA for the prevention, treatment, or cure of any medical condition, ailment, or disease. We must emphasize that any form of bodily introduction of these products into humans or animals is strictly prohibited by law. It is essential to adhere to these guidelines to ensure compliance with legal and ethical standards in research and experimentation.