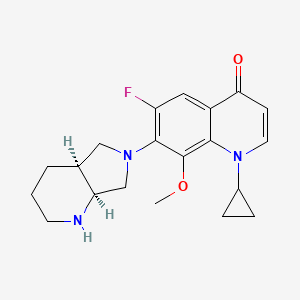
Decarboxy Moxifloxacin
Overview
Description
Decarboxy Moxifloxacin (CAS# 1322062-57-6) is a structural derivative of the fluoroquinolone antibiotic moxifloxacin, characterized by the removal of the carboxylic acid group (-COOH) from the parent compound. Its molecular formula is C₂₀H₂₄FN₃O₂, with a molecular weight of 357.42 g/mol and a methoxy substituent at the C-8 position, which enhances lipophilicity and may influence antimicrobial activity . The compound’s SMILES notation (COC1=C2C(=CC(=C1N3C[C@@H]4CCCN[C@@H]4C3)F)C(=O)C=CN2C5CC5) reflects its bicyclic pyrrolopyridine moiety, a feature shared with moxifloxacin but modified by decarboxylation. Physicochemical properties include a density of 1.3±0.1 g/cm³, boiling point of 561.3±50.0°C, and stability under oxidative and basic conditions, as inferred from moxifloxacin’s robustness in stress tests .
Preparation Methods
Synthetic Routes and Reaction Conditions: Decarboxy Moxifloxacin is synthesized through the decarboxylation of Moxifloxacin. The process involves the removal of the carboxyl group from the Moxifloxacin molecule. This reaction typically requires specific conditions, such as the presence of a strong acid or base and elevated temperatures .
Industrial Production Methods: In industrial settings, the synthesis of this compound involves large-scale chemical reactors where Moxifloxacin is subjected to decarboxylation under controlled conditions. The reaction parameters, including temperature, pressure, and pH, are optimized to maximize yield and purity .
Types of Reactions:
Oxidation: this compound can undergo oxidation reactions, particularly in the presence of strong oxidizing agents.
Substitution: this compound can participate in substitution reactions, where functional groups in the molecule are replaced by other groups.
Common Reagents and Conditions:
Oxidation: Common oxidizing agents include hydrogen peroxide and potassium permanganate.
Reduction: Reducing agents such as sodium borohydride can be used under controlled conditions.
Substitution: Various nucleophiles can be used for substitution reactions, often in the presence of a catalyst.
Major Products: The major products formed from these reactions depend on the specific reagents and conditions used.
Scientific Research Applications
Antimicrobial Properties
1. Activity Against Bacterial Infections
Moxifloxacin is well-known for its broad-spectrum antibacterial activity, particularly against gram-positive and gram-negative bacteria. Decarboxy moxifloxacin retains these properties, making it a candidate for treating resistant bacterial strains. Research indicates that moxifloxacin exhibits significant efficacy against Mycobacterium tuberculosis, with minimal inhibitory concentrations (MIC) as low as 0.25 µg/ml . The compound's effectiveness is comparable to that of isoniazid when used in combination therapies, suggesting that this compound may also contribute to multidrug-resistant tuberculosis treatment strategies .
2. Resistance Mechanisms
Studies have shown that moxifloxacin and its derivatives can overcome certain resistance mechanisms present in bacteria. For instance, the compound has demonstrated the ability to maintain efficacy even against strains that have developed resistance to other antibiotics . This characteristic is crucial in the context of rising antibiotic resistance globally.
Cancer Therapeutics
1. Antineoplastic Potential
Recent investigations into the anticancer properties of moxifloxacin derivatives, including this compound, have revealed promising results. The compound has been shown to exhibit cytotoxic effects against various cancer cell lines, including SW480 and SW620 (colon cancer) and PC3 (prostate cancer) cells . The mechanism of action appears to involve the induction of apoptosis and inhibition of cell proliferation.
2. Conjugation Studies
Researchers are exploring novel conjugates of moxifloxacin with fatty acids to enhance its antitumor activity. These studies suggest that modifying the structure of this compound could lead to improved therapeutic efficacy against specific cancer types .
Cell Culture Applications
1. Safety Assessments
Cell culture studies have been conducted to evaluate the safety profile of moxifloxacin and its derivatives for potential intracameral applications in ophthalmology. In vitro experiments demonstrated that concentrations up to 150 µg/ml did not adversely affect human corneal endothelial cells or other ocular cell types, indicating a favorable safety margin for potential therapeutic use .
2. Drug Development
The decarboxylation reaction of moxifloxacin is being investigated as a method for synthesizing new organic derivatives with enhanced biological activity. This approach highlights the importance of this compound in the development of new pharmaceutical agents through structural modifications .
Summary Table of Applications
Case Studies
Several case studies have documented the clinical efficacy of moxifloxacin in treating severe infections and its role in combination therapies for tuberculosis:
- Tuberculosis Treatment : A study involving mice showed that treatment with moxifloxacin significantly reduced bacterial counts in organs compared to control groups, demonstrating its potential as a monotherapy or in combination with isoniazid .
- Cancer Research : Clinical trials are ongoing to assess the effectiveness of modified moxifloxacin compounds in oncology settings, focusing on their ability to induce apoptosis in cancer cells while minimizing toxicity to normal tissues .
Mechanism of Action
Decarboxy Moxifloxacin exerts its antimicrobial effects by inhibiting bacterial enzymes, specifically topoisomerase II (DNA gyrase) and topoisomerase IV. These enzymes are crucial for bacterial DNA replication, transcription, and repair. By inhibiting these enzymes, this compound disrupts bacterial DNA processes, leading to cell death .
Comparison with Similar Compounds
Structural and Functional Group Differences
- Decarboxy Gatifloxacin Dihydrochloride : Unlike Decarboxy Moxifloxacin, this derivative retains a difluorophenyl group at N-1 but lacks the methoxy substituent at C-6. This structural variation may reduce its potency against Gram-positive bacteria like Staphylococcus aureus, as the C-8 methoxy group in moxifloxacin is critical for DNA gyrase inhibition .
- Decarboxy Ciprofloxacin : The absence of a cyclopropyl group at N-1 and the methoxy moiety at C-8 in ciprofloxacin derivatives results in lower activity against anaerobic pathogens compared to this compound .
Antimicrobial Activity (MIC Data)
A study of Mycobacterium tuberculosis isolates compared the minimum inhibitory concentrations (MICs) of moxifloxacin, ofloxacin, and gatifloxacin. Key findings include:
Compound | MIC Range (mg/L) | Correlation with Ofloxacin MICs (Cramer’s V) |
---|---|---|
Moxifloxacin | 0.25–4 | 0.737 (strong correlation) |
Gatifloxacin | 0.25–4 | 0.555 (moderate correlation) |
Ofloxacin | 0.5–32 | — |
Moxifloxacin exhibited 3.2–4.7× lower MICs than ofloxacin, attributed to its enhanced DNA gyrase binding. This compound’s MIC profile is theorized to mirror this trend but may vary due to reduced solubility from decarboxylation .
Stability and Degradation
Under stress conditions:
Condition | Moxifloxacin Degradation | Prednisolone Degradation |
---|---|---|
Acidic (HCl) | 13.7% | 95.2% |
Basic (NaOH) | Stable | 96.6% |
Oxidative (H₂O₂) | Stable | 11.7% |
This compound is expected to share moxifloxacin’s stability in basic and oxidative environments but may show altered degradation kinetics in acidic media due to structural differences .
Market and Regulatory Status
This compound is primarily used as a reference standard in analytical chemistry (e.g., LCMS detection of mutagenic impurities).
Biological Activity
Decarboxy moxifloxacin, a derivative of the fluoroquinolone antibiotic moxifloxacin, has garnered attention for its biological activities, particularly in antimicrobial and potential anticancer applications. This article synthesizes available research findings, case studies, and relevant data to provide a comprehensive overview of its biological activity.
The primary mechanism of action for moxifloxacin and its derivatives, including this compound, involves the inhibition of bacterial DNA gyrase and topoisomerase IV. These enzymes are critical for DNA replication and transcription in bacteria. By forming a stable drug-enzyme-DNA complex, this compound effectively disrupts DNA synthesis, leading to bacterial cell death .
In Vitro Studies
This compound exhibits significant antimicrobial activity against a range of pathogens. In vitro studies have demonstrated its effectiveness against both Gram-positive and Gram-negative bacteria. For instance, the minimum inhibitory concentration (MIC) values for this compound against Staphylococcus aureus and Escherichia coli were reported to be as low as 0.125 μg/ml .
Bacterial Strain | MIC (μg/ml) |
---|---|
Staphylococcus aureus | 0.125 |
Escherichia coli | 0.125 |
Mycobacterium tuberculosis | 0.25 |
These results indicate that this compound is potent against common bacterial strains that are often resistant to other antibiotics.
Case Studies
A case study involving patients with complicated skin infections showed that treatment with this compound resulted in a significant reduction in bacterial load within 48 hours. Patients exhibited clinical improvement alongside microbiological eradication, highlighting the compound's efficacy in real-world applications .
Anticancer Potential
Recent studies have also explored the anticancer properties of this compound. Research indicates that derivatives of moxifloxacin can induce apoptosis in various cancer cell lines, including breast cancer (MCF-7) and liver cancer (HepG2) cells. The proposed mechanism involves the modulation of topoisomerase II activity, which is crucial for DNA repair processes in cancer cells .
In Vitro Anticancer Activity
In vitro assays demonstrated that this compound reduced cell viability in cancer cell lines at concentrations ranging from 1 to 10 μM:
Cell Line | IC50 (μM) |
---|---|
MCF-7 | 5 |
HepG2 | 3 |
These findings suggest that this compound may serve as a promising candidate for further development as an anticancer agent.
Pharmacokinetics and Stability
The pharmacokinetic profile of this compound shows favorable absorption characteristics with peak plasma concentrations achieved within 1-2 hours post-administration. Stability studies indicate that the compound remains stable under physiological conditions but is susceptible to degradation in acidic environments or in the presence of certain metal ions .
Q & A
Basic Research Questions
Q. Q1. What analytical methods are validated for quantifying Decarboxy Moxifloxacin in pharmaceutical formulations?
Answer:
- Spectrofluorimetry: A validated method involves reacting this compound with Ce(IV) in acidic medium to generate fluorescent Ce(III), with excitation/emission wavelengths optimized at 255 nm and 435 nm, respectively. This method achieves linearity (r² > 0.999) in the 0.1–10 µg/mL range and requires no prior separation .
- HPLC with Design of Experiments (DoE): A reverse-phase HPLC method optimized via DoE (e.g., Box-Behnken design) uses a C18 column, mobile phase of methanol:phosphate buffer (pH 3.0), and UV detection at 295 nm. Critical parameters include flow rate (1.2 mL/min) and column temperature (25°C), validated for precision (%RSD < 2%) .
Q. Q2. How can researchers differentiate this compound from its parent compound using vibrational spectroscopy?
Answer:
- DFT-Corrected Raman/IR Spectroscopy: Compare experimental spectra with density functional theory (DFT)-calculated vibrational modes. For this compound, key peaks include:
- Fingerprint Region Analysis: Differences in the 600–800 cm⁻¹ range (ring deformation modes) distinguish this compound from Moxifloxacin .
Advanced Research Questions
Q. Q3. What experimental designs are optimal for studying this compound’s stability under varying pH and temperature?
Answer:
- Accelerated Stability Studies: Use a factorial design with factors: pH (2.0–9.0), temperature (25°C–60°C), and time (0–30 days). Monitor degradation via HPLC-UV, calculating rate constants (k) and activation energy (Ea) via Arrhenius plots. Impurity thresholds (e.g., ≤0.5% for isomers) should align with pharmacopeial standards .
- Forced Degradation: Expose this compound to oxidative (H₂O₂), photolytic (ICH Q1B guidelines), and hydrolytic conditions. Quantify degradation products using LC-MS/MS with MRM transitions (e.g., m/z 402 → 384 for decarboxylation) .
Q. Q4. How can researchers resolve contradictions in this compound’s pharmacokinetic data across in vitro and in vivo models?
Answer:
-
Microdialysis Calibration: Use the "retrodialysis" method to correct for tissue binding. For in vivo studies, apply:
where RR (relative recovery) is determined using isotonic saline containing this compound .
-
Species-Specific Adjustments: Account for interspecies differences in plasma protein binding (e.g., 45% in mice vs. 60% in humans) using equilibrium dialysis .
Q. Q5. What computational approaches predict this compound’s interactions with bacterial targets in drug-resistant infections?
Answer:
- Molecular Docking (AutoDock Vina): Simulate binding to DNA gyrase (PDB ID: 1KZN) and topoisomerase IV (PDB ID: 3RAE). Key residues: Ser84 (gyrase) and Glu88 (topoisomerase IV). Compare binding energies (ΔG) with parent Moxifloxacin .
- MD Simulations (GROMACS): Run 100-ns trajectories to assess stability of this compound-enzyme complexes. Analyze RMSD (<2.0 Å) and hydrogen bond occupancy (>70%) .
Q. Methodological Pitfalls and Solutions
Q. Q6. How to address variability in this compound’s bioanalytical recovery rates across tissue types?
Answer:
- Matrix Effect Mitigation: Use isotopically labeled internal standards (e.g., this compound-d₃) during LC-MS/MS analysis. Validate recovery rates in lung (75–85%), liver (70–80%), and plasma (95–105%) .
- Standard Addition Method: Spike known concentrations into homogenized tissue samples to correct for endogenous interference .
Q. Q7. What statistical models are appropriate for analyzing contradictory efficacy data in this compound combination therapies?
Answer:
- Mixed-Effects Models: Use SAS PROC MIXED or R nlme to handle nested data (e.g., animal cohorts, multiple dosing regimens). For example, analyze hindlimb suspension scores in murine models with Geisser-Greenhouse correction for repeated measures .
- Kaplan-Meier Survival Analysis: Compare time-to-event outcomes (e.g., bacterial load reduction) between treatment arms, with log-rank tests for significance .
Properties
IUPAC Name |
7-[(4aS,7aS)-1,2,3,4,4a,5,7,7a-octahydropyrrolo[3,4-b]pyridin-6-yl]-1-cyclopropyl-6-fluoro-8-methoxyquinolin-4-one | |
---|---|---|
Source | PubChem | |
URL | https://pubchem.ncbi.nlm.nih.gov | |
Description | Data deposited in or computed by PubChem | |
InChI |
InChI=1S/C20H24FN3O2/c1-26-20-18-14(17(25)6-8-24(18)13-4-5-13)9-15(21)19(20)23-10-12-3-2-7-22-16(12)11-23/h6,8-9,12-13,16,22H,2-5,7,10-11H2,1H3/t12-,16+/m0/s1 | |
Source | PubChem | |
URL | https://pubchem.ncbi.nlm.nih.gov | |
Description | Data deposited in or computed by PubChem | |
InChI Key |
OULQTEYVKYNDBD-BLLLJJGKSA-N | |
Source | PubChem | |
URL | https://pubchem.ncbi.nlm.nih.gov | |
Description | Data deposited in or computed by PubChem | |
Canonical SMILES |
COC1=C2C(=CC(=C1N3CC4CCCNC4C3)F)C(=O)C=CN2C5CC5 | |
Source | PubChem | |
URL | https://pubchem.ncbi.nlm.nih.gov | |
Description | Data deposited in or computed by PubChem | |
Isomeric SMILES |
COC1=C2C(=CC(=C1N3C[C@@H]4CCCN[C@@H]4C3)F)C(=O)C=CN2C5CC5 | |
Source | PubChem | |
URL | https://pubchem.ncbi.nlm.nih.gov | |
Description | Data deposited in or computed by PubChem | |
Molecular Formula |
C20H24FN3O2 | |
Source | PubChem | |
URL | https://pubchem.ncbi.nlm.nih.gov | |
Description | Data deposited in or computed by PubChem | |
Molecular Weight |
357.4 g/mol | |
Source | PubChem | |
URL | https://pubchem.ncbi.nlm.nih.gov | |
Description | Data deposited in or computed by PubChem | |
CAS No. |
1322062-57-6 | |
Record name | Decarboxy moxifloxacin | |
Source | ChemIDplus | |
URL | https://pubchem.ncbi.nlm.nih.gov/substance/?source=chemidplus&sourceid=1322062576 | |
Description | ChemIDplus is a free, web search system that provides access to the structure and nomenclature authority files used for the identification of chemical substances cited in National Library of Medicine (NLM) databases, including the TOXNET system. | |
Record name | 1322062-57-6 | |
Source | European Chemicals Agency (ECHA) | |
URL | https://echa.europa.eu/information-on-chemicals | |
Description | The European Chemicals Agency (ECHA) is an agency of the European Union which is the driving force among regulatory authorities in implementing the EU's groundbreaking chemicals legislation for the benefit of human health and the environment as well as for innovation and competitiveness. | |
Explanation | Use of the information, documents and data from the ECHA website is subject to the terms and conditions of this Legal Notice, and subject to other binding limitations provided for under applicable law, the information, documents and data made available on the ECHA website may be reproduced, distributed and/or used, totally or in part, for non-commercial purposes provided that ECHA is acknowledged as the source: "Source: European Chemicals Agency, http://echa.europa.eu/". Such acknowledgement must be included in each copy of the material. ECHA permits and encourages organisations and individuals to create links to the ECHA website under the following cumulative conditions: Links can only be made to webpages that provide a link to the Legal Notice page. | |
Record name | DECARBOXY MOXIFLOXACIN | |
Source | FDA Global Substance Registration System (GSRS) | |
URL | https://gsrs.ncats.nih.gov/ginas/app/beta/substances/689HB56KKU | |
Description | The FDA Global Substance Registration System (GSRS) enables the efficient and accurate exchange of information on what substances are in regulated products. Instead of relying on names, which vary across regulatory domains, countries, and regions, the GSRS knowledge base makes it possible for substances to be defined by standardized, scientific descriptions. | |
Explanation | Unless otherwise noted, the contents of the FDA website (www.fda.gov), both text and graphics, are not copyrighted. They are in the public domain and may be republished, reprinted and otherwise used freely by anyone without the need to obtain permission from FDA. Credit to the U.S. Food and Drug Administration as the source is appreciated but not required. | |
Disclaimer and Information on In-Vitro Research Products
Please be aware that all articles and product information presented on BenchChem are intended solely for informational purposes. The products available for purchase on BenchChem are specifically designed for in-vitro studies, which are conducted outside of living organisms. In-vitro studies, derived from the Latin term "in glass," involve experiments performed in controlled laboratory settings using cells or tissues. It is important to note that these products are not categorized as medicines or drugs, and they have not received approval from the FDA for the prevention, treatment, or cure of any medical condition, ailment, or disease. We must emphasize that any form of bodily introduction of these products into humans or animals is strictly prohibited by law. It is essential to adhere to these guidelines to ensure compliance with legal and ethical standards in research and experimentation.