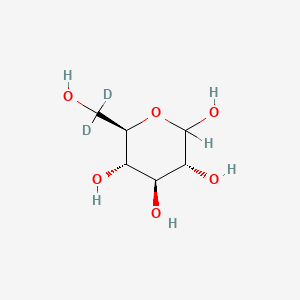
D-Glucose-6,6-d2
- Click on QUICK INQUIRY to receive a quote from our team of experts.
- With the quality product at a COMPETITIVE price, you can focus more on your research.
Overview
Description
Mechanism of Action
Target of Action
D-Glucose-6,6-d2, also known as Deuterated Glucose or Dextrose-6,6-d2 , primarily targets enzymes involved in the glycolytic pathway . These enzymes include hexokinase, phosphofructokinase 1 (PFK 1), and pyruvate kinase . These enzymes play a crucial role in the conversion of glucose to pyruvate, providing the cell with ATP under anaerobic conditions and supplying precursors for the biosynthesis of proteins, lipids, nucleic acids, and polysaccharides .
Mode of Action
The mode of action of this compound involves its interaction with the aforementioned enzymes. It is phosphorylated by hexokinase, a step necessary for its activity . The compound then undergoes a series of reactions facilitated by the enzymes in the glycolytic pathway .
Biochemical Pathways
This compound is involved in the glycolytic pathway, also known as the Meyerhoff, Embden, Parnas pathway . This pathway is ubiquitous in nature and is responsible for the anaerobic conversion of glucose to pyruvic acid . The glycolytic pathway also provides the cell with ATP under anaerobic conditions and supplies precursors for the biosynthesis of proteins, lipids, nucleic acids, and polysaccharides .
Result of Action
The result of the action of this compound is the production of pyruvate through the glycolytic pathway . This process provides the cell with ATP under anaerobic conditions and supplies precursors for the biosynthesis of proteins, lipids, nucleic acids, and polysaccharides .
Action Environment
The action environment of this compound is primarily the cell cytoplasm, where the enzymes of the glycolytic pathway are located
Biochemical Analysis
Biochemical Properties
D-Glucose-6,6-d2 participates in various biochemical reactions similar to its non-isotope labeled counterpart, glucose. Upon entering the cell, it is phosphorylated by hexokinase to form glucose-6-phosphate . This is a critical step in both glycolysis and the pentose phosphate pathway . The enzymes and proteins it interacts with include hexokinase, glucose-6-phosphate dehydrogenase, and phosphoglucose isomerase .
Cellular Effects
The effects of this compound on cells are largely similar to those of glucose. It plays a crucial role in cellular metabolism, contributing to the production of ATP through glycolysis and the pentose phosphate pathway . It also influences cell signaling pathways and gene expression, particularly those related to energy metabolism .
Molecular Mechanism
The molecular mechanism of this compound involves its conversion to glucose-6-phosphate by hexokinase, which is then used in various metabolic pathways . It can bind to and inhibit hexokinase, affecting glucose metabolism . Changes in gene expression may occur as a result of shifts in metabolic flux caused by the presence of this compound .
Dosage Effects in Animal Models
The effects of this compound at different dosages in animal models have not been extensively studied. It is reasonable to assume that, like glucose, its effects would vary with dosage, potentially influencing factors such as insulin sensitivity, energy metabolism, and possibly exerting toxic effects at high doses .
Metabolic Pathways
This compound is involved in several key metabolic pathways, including glycolysis, the pentose phosphate pathway, and glycogenesis . It interacts with enzymes such as hexokinase, glucose-6-phosphate dehydrogenase, and phosphoglucose isomerase .
Transport and Distribution
This compound is transported into cells via glucose transporters, such as those in the GLUT or SLC2A families . Once inside the cell, it is distributed within the cytoplasm and can be utilized in various metabolic pathways .
Subcellular Localization
The subcellular localization of this compound would be expected to be similar to that of glucose. After its conversion to glucose-6-phosphate, it can be found in the cytoplasm where glycolysis occurs, and in the endoplasmic reticulum and Golgi apparatus where it is involved in protein glycosylation .
Preparation Methods
Synthetic Routes and Reaction Conditions
The synthesis of D-Glucose-6,6-d2 typically involves the deuteration of glucose. One common method is the catalytic exchange reaction, where glucose is treated with deuterium oxide (D2O) in the presence of a catalyst. This process replaces the hydrogen atoms with deuterium atoms at specific positions .
Industrial Production Methods
Industrial production of this compound follows similar principles but on a larger scale. The process involves the use of high-purity deuterium oxide and advanced catalytic systems to ensure efficient and selective deuteration. The final product is then purified to achieve the desired isotopic purity and chemical purity .
Chemical Reactions Analysis
Types of Reactions
D-Glucose-6,6-d2 undergoes various chemical reactions similar to those of regular glucose, including:
Oxidation: this compound can be oxidized to form gluconic acid or glucuronic acid.
Reduction: It can be reduced to form sorbitol.
Substitution: The hydroxyl groups in this compound can be substituted with other functional groups.
Common Reagents and Conditions
Oxidation: Common oxidizing agents include nitric acid and bromine water.
Reduction: Sodium borohydride or hydrogen gas in the presence of a catalyst can be used for reduction reactions.
Substitution: Various reagents such as acetic anhydride or benzoyl chloride can be used for substitution reactions.
Major Products Formed
Oxidation: Gluconic acid, glucuronic acid.
Reduction: Sorbitol.
Substitution: Acetylated or benzoylated glucose derivatives.
Scientific Research Applications
D-Glucose-6,6-d2 is widely used in scientific research due to its isotopic labeling, which allows for precise tracking and analysis in various fields:
Chemistry: Used in nuclear magnetic resonance (NMR) spectroscopy to study glucose metabolism and molecular interactions.
Biology: Employed in metabolic studies to trace glucose pathways and understand cellular processes.
Medicine: Utilized in clinical studies to investigate glucose metabolism in diseases such as diabetes and cancer.
Industry: Applied in the development of new pharmaceuticals and in quality control processes
Comparison with Similar Compounds
Similar Compounds
D-Glucose-1,2,3,4,5,6,6-d7: A fully deuterated glucose molecule.
D-Glucose-13C6: Glucose labeled with carbon-13 isotopes.
D-Glucose-1-13C: Glucose labeled with carbon-13 at the first position.
Uniqueness
D-Glucose-6,6-d2 is unique due to its selective deuteration at the sixth position, which provides specific insights into metabolic processes involving this part of the glucose molecule. This selective labeling allows researchers to study the effects of deuterium substitution on glucose metabolism and to trace metabolic pathways with high precision .
Biological Activity
D-Glucose-6,6-d2, also known as deuterated glucose, is an isotopically labeled form of glucose that has garnered attention in various fields of biological research due to its unique properties and applications. This article will explore the biological activity of this compound, including its biochemical pathways, cellular effects, and research applications, supported by data tables and relevant case studies.
Chemical Structure and Properties
this compound retains the fundamental structure of glucose but features deuterium atoms at the sixth carbon position. This alteration allows researchers to track its metabolism in biological systems while participating in the same fundamental chemical reactions as its non-labeled counterpart.
Biochemical Pathways
Upon entering the cell, this compound is phosphorylated by hexokinase to form glucose-6-phosphate. This initial step is crucial for its participation in the glycolytic pathway, gluconeogenesis, and glycogen synthesis. The deuterium labeling can influence reaction kinetics due to kinetic isotope effects, making it a valuable tool for studying metabolic pathways.
Cellular Effects
This compound exhibits cellular effects similar to those of glucose. It plays a crucial role in cellular metabolism by contributing to ATP production through glycolysis and the pentose phosphate pathway. The compound's incorporation into various metabolic pathways allows for detailed investigations into enzyme activity and substrate utilization.
Table 1: Comparison of Biological Activities
Activity Type | This compound | Non-labeled Glucose |
---|---|---|
Glycolysis | Participates as glucose substrate | Primary energy source |
Phosphorylation | Forms glucose-6-phosphate | Forms glucose-6-phosphate |
Kinetic Isotope Effects | Alters reaction rates | Standard reaction rates |
Metabolic Pathways | Glycolysis, gluconeogenesis | Glycolysis, gluconeogenesis |
Research Applications
This compound is widely used in scientific research due to its isotopic labeling capabilities. It allows for precise tracking and analysis in various fields:
- Metabolic Studies : It is employed to trace glucose pathways and understand cellular processes.
- Nuclear Magnetic Resonance (NMR) Spectroscopy : Used to study glucose metabolism and molecular interactions.
- Clinical Research : Investigates glucose metabolism in diseases such as diabetes and cancer .
Case Study: Cerebral Glucose Metabolism
A study assessed cerebral glucose metabolic rates using this compound in rats. The compound was infused intravenously at a dosage of 1.3 g/kg body weight. Following infusion, researchers monitored the dynamics of labeled glucose levels in the brain through dynamic magnetic resonance spectroscopy (DMRS). The results demonstrated that this compound was effectively transported into the brain and metabolized through glycolysis and oxidative phosphorylation pathways .
Properties
Key on ui mechanism of action |
Glucose supplies most of the energy to all tissues by generating energy molecules ATP and NADH during a series of metabolism reactions called glycolysis. Glycolysis can be divided into two main phases where the preparatory phase is initiated by the phosphorylation of glucose by hexokinase to form glucose 6-phosphate. The addition of the high-energy phosphate group activates glucose for the subsequent breakdown in later steps of glycolysis and is the rate-limiting step. Products end up as substrates for following reactions, to ultimately convert C6 glucose molecule into two C3 sugar molecules. These products enter the energy-releasing phase where the total of 4ATP and 2NADH molecules are generated per one glucose molecule. The total aerobic metabolism of glucose can produce up to 36 ATP molecules. These energy-producing reactions of glucose are limited to D-glucose as L-glucose cannot be phosphorylated by hexokinase. Glucose can act as precursors to generate other biomolecules such as vitamin C. It plays a role as a signaling molecule to control glucose and energy homeostasis. Glucose can regulate gene transcription, enzyme activity, hormone secretion, and the activity of glucoregulatory neurons. The types, number, and kinetics of glucose transporters expressed depends on the tissues and fine-tunes glucose uptake, metabolism, and signal generation to preserve cellular and whole body metabolic integrity. |
---|---|
CAS No. |
18991-62-3 |
Molecular Formula |
C6H12O6 |
Molecular Weight |
182.17 g/mol |
IUPAC Name |
(2R,3S,4R,5R)-6,6-dideuterio-2,3,4,5,6-pentahydroxyhexanal |
InChI |
InChI=1S/C6H12O6/c7-1-3(9)5(11)6(12)4(10)2-8/h1,3-6,8-12H,2H2/t3-,4+,5+,6+/m0/s1/i2D2 |
InChI Key |
GZCGUPFRVQAUEE-MOLODFKWSA-N |
SMILES |
C(C1C(C(C(C(O1)O)O)O)O)O |
Isomeric SMILES |
[2H]C([2H])([C@H]([C@H]([C@@H]([C@H](C=O)O)O)O)O)O |
Canonical SMILES |
C(C(C(C(C(C=O)O)O)O)O)O |
physical_description |
Dry Powder; Liquid, Other Solid; NKRA; Liquid White crystalline powder; Odorless; [Acros Organics MSDS] |
solubility |
Soluble |
Synonyms |
D-Glucopyranose-d2; Glucopyranose-d2; NSC 287045-d2; |
Origin of Product |
United States |
Q1: What structural information about the oxidative cleavage of D-Glucose-6,6′-d2 can be gleaned from the research using NMR spectroscopy?
A1: The research utilizes deuterium labeling and NMR spectroscopy to elucidate the regiochemistry of oxidative glycol-scission reactions on D-Glucose-6,6′-d2. Specifically, the study demonstrates that oxidation of D-Glucose-6,6′-d2 with lead tetraacetate yields 2,3-di-O-formyl-D-erythrose-4,4′-d2. [] This finding, based on the observation of deuterium's effect on the NMR signal of adjacent protons, provides evidence that both C6 atoms of the original glucose molecule are retained in the erythrose product, indicating a specific cleavage pattern during the reaction.
Disclaimer and Information on In-Vitro Research Products
Please be aware that all articles and product information presented on BenchChem are intended solely for informational purposes. The products available for purchase on BenchChem are specifically designed for in-vitro studies, which are conducted outside of living organisms. In-vitro studies, derived from the Latin term "in glass," involve experiments performed in controlled laboratory settings using cells or tissues. It is important to note that these products are not categorized as medicines or drugs, and they have not received approval from the FDA for the prevention, treatment, or cure of any medical condition, ailment, or disease. We must emphasize that any form of bodily introduction of these products into humans or animals is strictly prohibited by law. It is essential to adhere to these guidelines to ensure compliance with legal and ethical standards in research and experimentation.