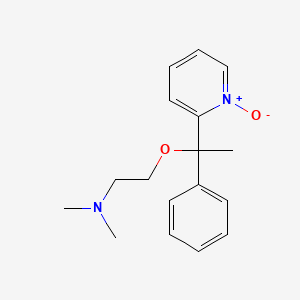
Doxylamine N-Oxide
- Click on QUICK INQUIRY to receive a quote from our team of experts.
- With the quality product at a COMPETITIVE price, you can focus more on your research.
Description
Doxylamine N-Oxide, also known as doxylamine succinate N-oxide, is a chemical compound that belongs to the family of antihistamines. It is a derivative of doxylamine, an antihistamine that is commonly used to treat allergies, insomnia, and nausea. This compound has gained attention in recent years due to its potential applications in scientific research.
Scientific Research Applications
Metabolite Analysis and Characterization : Doxylamine N-Oxide has been identified and characterized as a metabolite of doxylamine in several studies. For instance, Korfmacher et al. (1988) used thermospray mass spectrometry to analyze this compound and other metabolites, demonstrating the utility of this technique in metabolite analysis (Korfmacher et al., 1988).
Forensic Applications : María del Mar Ramírez Fernández et al. (2022) explored the incorporation of doxylamine and this compound in human hair. This study highlighted the relationship between dosage and hair concentration, and the impact of hair treatments like oxidative dying on these concentrations, which could have forensic implications (Fernández et al., 2022).
Metabolic Pathways in Animals : Slikker et al. (1986) investigated the metabolic fate of doxylamine in rhesus monkeys, identifying this compound among the metabolites. This study provided insights into the metabolic pathways and the role of this compound in these processes (Slikker et al., 1986).
Toxicological Studies : Bockholdt et al. (2001) reported on cases of doxylamine poisoning, where high concentrations of doxylamine and its metabolites, including this compound, were found in the blood and organs. This study contributes to the understanding of doxylamine's toxicological profile (Bockholdt et al., 2001).
Chemical and Pharmacological Properties : Studies like those by Oliveira et al. (2018) and Wunderlin et al. (2012) explore the broader chemical and pharmacological properties of compounds with N-O bonds, like this compound. These investigations are crucial for understanding the potential therapeutic applications and environmental impacts of these compounds (Oliveira et al., 2018); (Wunderlin et al., 2012).
Properties
{ "Design of the Synthesis Pathway": "The synthesis of Doxylamine N-Oxide can be achieved through the oxidation of Doxylamine using a suitable oxidizing agent.", "Starting Materials": [ "Doxylamine", "Oxidizing agent (e.g. hydrogen peroxide, peracetic acid, m-chloroperbenzoic acid)" ], "Reaction": [ "Doxylamine is dissolved in a suitable solvent (e.g. methanol, ethanol, acetonitrile)", "The oxidizing agent is added to the solution slowly with stirring at a temperature of 0-5°C", "The reaction mixture is allowed to stir for a suitable period of time (e.g. 2-4 hours) at the same temperature", "The reaction is quenched by adding a suitable quenching agent (e.g. sodium bisulfite, sodium thiosulfate)", "The mixture is filtered to remove any solid impurities", "The filtrate is concentrated under reduced pressure to obtain the crude product", "The crude product is purified by recrystallization or column chromatography using a suitable solvent system (e.g. methanol-chloroform)" ] } | |
97143-65-2 | |
Molecular Formula |
C17H22N2O2 |
Molecular Weight |
286.37 g/mol |
IUPAC Name |
N,N-dimethyl-2-(1-phenyl-1-pyridin-2-ylethoxy)ethanamine oxide |
InChI |
InChI=1S/C17H22N2O2/c1-17(15-9-5-4-6-10-15,16-11-7-8-12-18-16)21-14-13-19(2,3)20/h4-12H,13-14H2,1-3H3 |
InChI Key |
SSGKPGKMFJONCR-UHFFFAOYSA-N |
SMILES |
CC(C1=CC=CC=C1)(C2=CC=CC=[N+]2[O-])OCCN(C)C |
Canonical SMILES |
CC(C1=CC=CC=C1)(C2=CC=CC=N2)OCC[N+](C)(C)[O-] |
synonyms |
N,N-Dimethyl-2-[1-phenyl-1-(2-pyridinyl)ethoxy]ethanamine N-Oxide; |
Origin of Product |
United States |
Q1: What are the major metabolic pathways of doxylamine in primates?
A1: Research using rhesus monkeys [] identified four primary metabolic pathways for doxylamine:
Q2: How does the metabolism of doxylamine differ between rats and primates?
A2: While both species exhibit N-oxidation, N-demethylation, and side-chain cleavage, a key difference lies in the presence of ring-hydroxylated metabolites in rats [], which were not identified in rhesus monkeys []. This highlights potential species-specific variations in doxylamine metabolism.
Q3: What analytical techniques are most commonly used to identify and characterize doxylamine and its metabolites?
A3: A combination of techniques is employed, with a strong emphasis on mass spectrometry (MS) approaches. Key methods include:
- Chemical ionization mass spectrometry: Utilized with methane or ammonia as reagent gases for the identification of doxylamine and its metabolites [].
- Thermospray mass spectrometry (TSP/MS) and tandem mass spectrometry (TSP/MS/MS): Provide structural information and fragmentation patterns for doxylamine, doxylamine N-oxide, and other metabolites [].
- High-performance liquid chromatography (HPLC): Used in conjunction with MS for the separation and identification of doxylamine and its metabolites in biological samples [, ].
- Chemical derivatization techniques: Employed to modify doxylamine and its metabolites to facilitate their detection and identification by MS [, ].
Q4: Does doxylamine get incorporated into human hair, and if so, can cosmetic treatments affect its detection?
A4: Research has shown that both doxylamine and its metabolite, this compound, are incorporated into human hair []. Interestingly, the application of a permanent oxidative hair dye was found to increase the concentration of this compound detected in hair, while decreasing the concentration of doxylamine itself []. This finding highlights the potential impact of cosmetic treatments on drug analysis in hair samples.
Q5: What is the role of in vitro models in studying doxylamine metabolism?
A5: In vitro models, such as isolated rat hepatocytes [], provide valuable tools for investigating the metabolic pathways of doxylamine. Incubation of doxylamine with rat hepatocytes generated several metabolites observed in vivo, confirming the role of hepatic metabolism in the drug's elimination []. Furthermore, studies using human and rat intestinal microflora demonstrated that anaerobic bacteria were unable to degrade doxylamine [], suggesting a limited role for gut bacteria in its metabolism.
Disclaimer and Information on In-Vitro Research Products
Please be aware that all articles and product information presented on BenchChem are intended solely for informational purposes. The products available for purchase on BenchChem are specifically designed for in-vitro studies, which are conducted outside of living organisms. In-vitro studies, derived from the Latin term "in glass," involve experiments performed in controlled laboratory settings using cells or tissues. It is important to note that these products are not categorized as medicines or drugs, and they have not received approval from the FDA for the prevention, treatment, or cure of any medical condition, ailment, or disease. We must emphasize that any form of bodily introduction of these products into humans or animals is strictly prohibited by law. It is essential to adhere to these guidelines to ensure compliance with legal and ethical standards in research and experimentation.