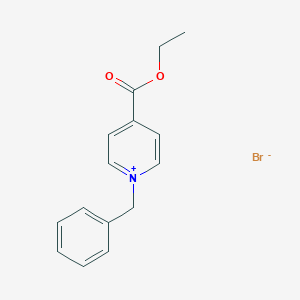
Pyridinium, 4-(ethoxycarbonyl)-1-(phenylmethyl)-, bromide
- Click on QUICK INQUIRY to receive a quote from our team of experts.
- With the quality product at a COMPETITIVE price, you can focus more on your research.
Overview
Description
Pyridinium, 4-(ethoxycarbonyl)-1-(phenylmethyl)-, bromide, also known as this compound, is a useful research compound. Its molecular formula is C15H16BrNO2 and its molecular weight is 322.2 g/mol. The purity is usually 95%.
BenchChem offers high-quality this compound suitable for many research applications. Different packaging options are available to accommodate customers' requirements. Please inquire for more information about this compound including the price, delivery time, and more detailed information at info@benchchem.com.
Scientific Research Applications
Synthesis and Reactivity
Synthon for Functionalized Pyrrolidines : The compound has been used as a synthon to synthesize functionalized pyrrolidines and spiro-pyrrolidines, highlighting its utility in producing complex organic structures and potentially active pharmaceutical ingredients (Guideri, Noschese, & Ponticelli, 2012).
Photophysical Properties : It serves as a fluorophore for studying solvent-sensitive charge-transfer absorption behaviors and dual-emissive fluorescent properties, which can be essential in developing sensors and imaging agents (Li et al., 2009).
Corrosion Inhibition : Derivatives of this compound have been studied as corrosion inhibitors for mild steel in acidic environments, indicating its application in protecting metal surfaces and infrastructure (Raheem & Shihab, 2022).
Regioselective Oxidation : The compound has been involved in studies for the regioselective oxidation of pyridinium salts into 1H-pyridin-2-ones, showcasing its potential in organic synthesis and modification of molecules for various applications (Terán et al., 2000).
Liquid Crystal Formation : Research on pyridinium bromides with a biphenyl core, including derivatives similar to the compound , has shown that these molecules can form ionic liquid crystals. This property is useful in the development of display technologies and materials science (Cui, Sapagovas, & Lattermann, 2002).
Host-Guest Chemistry : Studies have also explored its role in forming stable ternary complexes in host-guest chemistry, a fundamental aspect of supramolecular chemistry that has implications in drug delivery systems and molecular recognition (Senler et al., 2012).
Mechanism of Action
Target of Action
Pyridinium salts, including Pyridinium, 4-(ethoxycarbonyl)-1-(phenylmethyl)-, bromide, are structurally diverse and are found in many natural products and bioactive pharmaceuticals . They have been used in a wide range of research topics due to their intriguing role . .
Mode of Action
The mode of action of pyridinium salts is diverse and depends on their structure and the specific targets they interact with. For instance, some pyridinium salts are known to act as anti-microbial, anti-cancer, anti-malarial, and anti-cholinesterase inhibitors . Pyridinium ylides, a class of pyridinium salts, are usually considered nucleophiles that can undergo various reactions involving electron pairs . .
Biochemical Pathways
The biochemical pathways affected by pyridinium salts are diverse and depend on their specific targets and mode of action. Some pyridinium salts have been reported to have importance in materials science and biological issues related to gene delivery . .
Properties
IUPAC Name |
ethyl 1-benzylpyridin-1-ium-4-carboxylate;bromide |
Source
|
---|---|---|
Source | PubChem | |
URL | https://pubchem.ncbi.nlm.nih.gov | |
Description | Data deposited in or computed by PubChem | |
InChI |
InChI=1S/C15H16NO2.BrH/c1-2-18-15(17)14-8-10-16(11-9-14)12-13-6-4-3-5-7-13;/h3-11H,2,12H2,1H3;1H/q+1;/p-1 |
Source
|
Source | PubChem | |
URL | https://pubchem.ncbi.nlm.nih.gov | |
Description | Data deposited in or computed by PubChem | |
InChI Key |
QDURSZTWSNZWLI-UHFFFAOYSA-M |
Source
|
Source | PubChem | |
URL | https://pubchem.ncbi.nlm.nih.gov | |
Description | Data deposited in or computed by PubChem | |
Canonical SMILES |
CCOC(=O)C1=CC=[N+](C=C1)CC2=CC=CC=C2.[Br-] |
Source
|
Source | PubChem | |
URL | https://pubchem.ncbi.nlm.nih.gov | |
Description | Data deposited in or computed by PubChem | |
Molecular Formula |
C15H16BrNO2 |
Source
|
Source | PubChem | |
URL | https://pubchem.ncbi.nlm.nih.gov | |
Description | Data deposited in or computed by PubChem | |
DSSTOX Substance ID |
DTXSID00441893 |
Source
|
Record name | Pyridinium, 4-(ethoxycarbonyl)-1-(phenylmethyl)-, bromide | |
Source | EPA DSSTox | |
URL | https://comptox.epa.gov/dashboard/DTXSID00441893 | |
Description | DSSTox provides a high quality public chemistry resource for supporting improved predictive toxicology. | |
Molecular Weight |
322.20 g/mol |
Source
|
Source | PubChem | |
URL | https://pubchem.ncbi.nlm.nih.gov | |
Description | Data deposited in or computed by PubChem | |
CAS No. |
23019-61-6 |
Source
|
Record name | Pyridinium, 4-(ethoxycarbonyl)-1-(phenylmethyl)-, bromide | |
Source | EPA DSSTox | |
URL | https://comptox.epa.gov/dashboard/DTXSID00441893 | |
Description | DSSTox provides a high quality public chemistry resource for supporting improved predictive toxicology. | |
Disclaimer and Information on In-Vitro Research Products
Please be aware that all articles and product information presented on BenchChem are intended solely for informational purposes. The products available for purchase on BenchChem are specifically designed for in-vitro studies, which are conducted outside of living organisms. In-vitro studies, derived from the Latin term "in glass," involve experiments performed in controlled laboratory settings using cells or tissues. It is important to note that these products are not categorized as medicines or drugs, and they have not received approval from the FDA for the prevention, treatment, or cure of any medical condition, ailment, or disease. We must emphasize that any form of bodily introduction of these products into humans or animals is strictly prohibited by law. It is essential to adhere to these guidelines to ensure compliance with legal and ethical standards in research and experimentation.