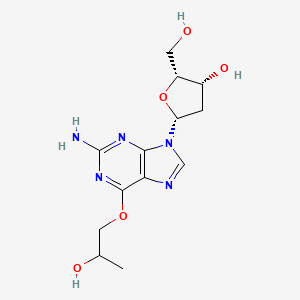
O6-(2-Hydroxypropyl)-2'-deoxyguanosine
Overview
Description
O6-(2-Hydroxypropyl)-2’-deoxyguanosine is a modified nucleoside that has garnered significant interest in scientific research due to its unique structural and chemical properties. This compound is derived from 2’-deoxyguanosine, a nucleoside component of DNA, and features a hydroxypropyl group attached to the oxygen atom at the sixth position of the guanine base. This modification can influence the compound’s interactions with other molecules, making it a valuable tool in various fields of study.
Preparation Methods
Synthetic Routes and Reaction Conditions
The synthesis of O6-(2-Hydroxypropyl)-2’-deoxyguanosine typically involves the alkylation of 2’-deoxyguanosine. One common method is to react 2’-deoxyguanosine with 2-bromo-1-propanol under basic conditions. The reaction is usually carried out in an organic solvent such as dimethyl sulfoxide (DMSO) or acetonitrile, with a base like potassium carbonate or sodium hydroxide to facilitate the nucleophilic substitution reaction. The reaction mixture is then purified using chromatographic techniques to isolate the desired product.
Industrial Production Methods
While specific industrial production methods for O6-(2-Hydroxypropyl)-2’-deoxyguanosine are not well-documented, the general principles of nucleoside modification can be applied. Large-scale production would likely involve optimizing the reaction conditions to maximize yield and purity, using automated synthesis equipment, and employing high-performance liquid chromatography (HPLC) for purification.
Chemical Reactions Analysis
Types of Reactions
O6-(2-Hydroxypropyl)-2’-deoxyguanosine can undergo various chemical reactions, including:
Oxidation: The hydroxypropyl group can be oxidized to form aldehyde or carboxylic acid derivatives.
Reduction: The compound can be reduced to modify the hydroxypropyl group, potentially forming alcohol derivatives.
Substitution: The hydroxypropyl group can be substituted with other functional groups through nucleophilic substitution reactions.
Common Reagents and Conditions
Oxidation: Reagents such as sodium hypochlorite or TEMPO (2,2,6,6-tetramethylpiperidine-1-oxyl) can be used under mild conditions to oxidize the hydroxypropyl group.
Reduction: Reducing agents like sodium borohydride or lithium aluminum hydride can be employed to reduce the hydroxypropyl group.
Substitution: Nucleophiles such as thiols or amines can be used in the presence of a base to substitute the hydroxypropyl group.
Major Products Formed
Oxidation: Aldehyde or carboxylic acid derivatives.
Reduction: Alcohol derivatives.
Substitution: Various substituted derivatives depending on the nucleophile used.
Scientific Research Applications
O6-(2-Hydroxypropyl)-2’-deoxyguanosine has several scientific research applications, including:
Chemistry: Used as a model compound to study nucleoside modifications and their effects on DNA structure and function.
Biology: Employed in studies of DNA repair mechanisms and the effects of DNA damage.
Medicine: Investigated for its potential role in cancer research, particularly in understanding how modified nucleosides can influence mutagenesis and carcinogenesis.
Industry: Utilized in the development of nucleoside analogs for therapeutic applications, including antiviral and anticancer drugs.
Mechanism of Action
The mechanism of action of O6-(2-Hydroxypropyl)-2’-deoxyguanosine involves its incorporation into DNA, where it can affect the DNA’s structural integrity and function. The hydroxypropyl group can form hydrogen bonds and other interactions with surrounding molecules, potentially influencing DNA replication and repair processes. This compound can also serve as a substrate for DNA polymerases and other enzymes involved in nucleic acid metabolism, thereby affecting cellular processes at the molecular level.
Comparison with Similar Compounds
Similar Compounds
O6-Methyl-2’-deoxyguanosine: Another modified nucleoside with a methyl group at the sixth position of guanine.
O6-Ethyl-2’-deoxyguanosine: Features an ethyl group at the sixth position of guanine.
O6-Benzyl-2’-deoxyguanosine: Contains a benzyl group at the sixth position of guanine.
Uniqueness
O6-(2-Hydroxypropyl)-2’-deoxyguanosine is unique due to the presence of the hydroxypropyl group, which can engage in additional hydrogen bonding and other interactions compared to simpler alkyl groups. This can lead to distinct effects on DNA structure and function, making it a valuable tool for studying nucleoside modifications and their biological implications.
Biological Activity
O6-(2-Hydroxypropyl)-2'-deoxyguanosine (OHPdG) is a modified nucleoside that has garnered interest due to its biological activity, particularly in the context of DNA damage and repair mechanisms. This article compiles research findings, case studies, and data tables to elucidate the biological implications of OHPdG.
Chemical Structure and Properties
OHPdG is an alkylated derivative of 2'-deoxyguanosine, where a 2-hydroxypropyl group is attached to the O6 position of the guanine base. This modification can lead to various biological effects, including mutagenicity and alterations in DNA repair processes.
Biological Activity Overview
The biological activity of OHPdG primarily revolves around its role as a DNA adduct. The formation of such adducts can interfere with normal DNA replication and transcription, potentially leading to mutations and cancer development.
- DNA Damage : OHPdG can form stable adducts with DNA, which may lead to mispairing during replication. This mispairing can result in G:C to A:T transitions, contributing to mutagenesis.
- Interference with DNA Repair : The presence of OHPdG in DNA can inhibit the activity of repair enzymes such as O6-alkylguanine-DNA alkyltransferase (AGT), which is crucial for the removal of alkyl groups from the O6 position of guanine residues. This inhibition can lead to an accumulation of DNA damage.
- Cellular Response : Cells containing OHPdG-modified DNA may activate stress response pathways, including apoptosis or senescence, depending on the extent of damage and cellular context.
Case Studies and Experimental Data
Several studies have investigated the formation and persistence of OHPdG in biological systems:
- Study on Rat Models : A study demonstrated that following administration of N-nitroso-N-(2-hydroxypropyl)urea (HPU), significant levels of OHPdG were detected in the liver and kidney tissues. The concentration peaked at approximately 81 μmol OHPdG/mol deoxyguanosine in the kidneys within 2 hours post-administration, indicating rapid formation after exposure .
- Repair Kinetics : The repair kinetics of OHPdG were assessed in various tissues. In liver tissue, the half-life for repair was approximately 12 hours, while in kidney and lung tissues it was longer (40 hours and 48 hours respectively). Notably, cerebral tissue exhibited a slower repair rate with 62% of initial OHPdG persisting for up to 7 days .
- Mutagenicity Assessment : In vitro assays have shown that cells exposed to OHPdG exhibit increased mutagenic rates compared to controls. This was particularly evident in sensitive cell lines where replication errors led to significant G:C to A:T transitions .
Data Table: Summary of Key Findings
Q & A
Q. Basic: What methodologies are employed to detect and quantify O6-(2-Hydroxypropyl)-2'-deoxyguanosine in DNA adduct studies?
Answer:
The most advanced method involves liquid chromatography-nanoelectrospray ionization high-resolution tandem mass spectrometry (LC-NSI-HRMS/MS) . This technique uses a gradient elution (98% 5 mM NH4OAc, 2% CH3CN) on a nano-HPLC column, with precursor ions isolated via quadrupole and fragmented via high-energy collision dissociation (HCD). Detection occurs via Orbitrap at 120,000 resolution, enabling accurate mass analysis (±15 ppm tolerance). Validation includes spiking known quantities of the adduct into DNA, generating calibration curves (6.5–422 amol on-column), and determining limits of detection (LOD: 6.5 amol) and quantification (LOQ: 100 amol) . Compared to low-resolution LC-ESI-MS/MS, this method reduces background noise and improves sensitivity by >10-fold, critical for detecting adducts in tissues like esophageal mucosa .
Q. Advanced: How do enantiomeric forms of N′-nitrosonornicotine (NNN) influence the formation of this compound in vivo?
Answer:
Enantiomer-specific metabolic activation drives synergistic adduct formation. In rats treated with racemic NNN (28–56 ppm), esophageal O6-POB-dGuo levels exceeded the sum of individual (R)-NNN and (S)-NNN treatments, suggesting (R)-NNN enhances (S)-NNN-derived adducts . However, this synergy is dose-dependent: observed at low/mid doses but absent at high doses. Tissue specificity is critical—synergy occurs in esophageal/oral mucosa but not in liver or lung . Mechanistically, (R)-NNN may increase activation of (S)-NNN metabolites or inhibit repair enzymes like O6-alkylguanine-DNA alkyltransferase (AGT) .
Q. Basic: What analytical challenges arise when quantifying DNA adducts like this compound in complex biological matrices?
Answer:
Key challenges include:
- Low abundance : Adducts often occur at 1–100 adducts per 10^8 nucleotides, necessitating ultrasensitive detection .
- Matrix interference : Co-eluting biomolecules (e.g., unmodified nucleosides) obscure signals. High-resolution mass spectrometry mitigates this by isolating exact masses (e.g., m/z 454.1705 for O6-POB-dGuo) .
- Validation rigor : Accuracy (102% ± 9%) and precision (CV <10%) require spiked recovery experiments and isotope-labeled internal standards (e.g., [pyridine-D4]O6-POB-dGuo) .
Q. Advanced: How does tissue-specific repair enzyme activity modulate this compound persistence?
Answer:
AGT variants exhibit tissue-specific efficiency. In rat nasal olfactory mucosa, AGT repairs O6-POB-dGuo rapidly, resulting in lower adduct retention compared to esophageal mucosa, where AGT activity is lower. This explains why adduct levels in the esophagus are 3–5× higher than in nasal tissues after NNN exposure . Additionally, AGT inhibition by aldehydes (e.g., acetaldehyde) exacerbates adduct accumulation in alcohol-associated carcinogenesis models .
Q. Basic: What synthetic strategies enable the preparation of O6-modified 2′-deoxyguanosine derivatives for mechanistic studies?
Answer:
Pd-catalyzed cross-coupling of O6-arylsulfonates with arylboronic acids is a robust method. For example, O6-(2-mesitylenesulfonyl)-2′-deoxyguanosine reacts with boronic acids under Pd(0) catalysis to yield 2-amino-6-arylpurine deoxynucleosides. Key steps include sulfonate activation of the O6 position and optimizing coupling conditions (e.g., solvent, temperature) to achieve >80% yields .
Q. Advanced: Why do this compound adduct levels vary across tissues despite systemic carcinogen exposure?
Answer:
Variation arises from:
- Metabolic activation : Cytochrome P450 isoforms (e.g., CYP2A13 in nasal mucosa) bioactivate NNN to DNA-reactive species preferentially in certain tissues .
- Adduct stability : O6-POB-dGuo is more persistent in tissues with low AGT activity (e.g., esophagus) versus high-activity tissues (e.g., liver) .
- Dosage thresholds : In lung tissue, adducts are detectable only above 28 ppm NNN, highlighting tissue-specific susceptibility .
Q. Basic: How are this compound adducts used as biomarkers in toxicology studies?
Answer:
These adducts serve as biomarkers of alkylating agent exposure (e.g., tobacco-specific nitrosamines). Quantification via LC-NSI-HRMS/MS in rat models links adduct levels to tumorigenic outcomes, with esophageal O6-POB-dGuo correlating with squamous carcinoma incidence . In humans, analogous adducts (e.g., O6-(2-hydroxyethyl)-2′-deoxyguanosine) are proposed biomarkers for ethylene oxide genotoxicity .
Q. Advanced: What experimental models best recapitulate in vivo adduct formation and repair dynamics?
Answer:
- In vivo rat models : Mimic metabolic heterogeneity and tissue-specific repair. For example, racemic NNN dosing in F344 rats replicates human tobacco exposure .
- In vitro AGT assays : Recombinant AGT incubated with synthetic O6-POB-dGuo quantifies repair kinetics (e.g., kcat/Km values) .
- Organoid cultures : Primary esophageal epithelial organoids retain tissue-specific metabolism and repair enzyme profiles, enabling mechanistic studies without systemic variability .
Properties
IUPAC Name |
(2R,3R,5R)-5-[2-amino-6-(2-hydroxypropoxy)purin-9-yl]-2-(hydroxymethyl)oxolan-3-ol | |
---|---|---|
Source | PubChem | |
URL | https://pubchem.ncbi.nlm.nih.gov | |
Description | Data deposited in or computed by PubChem | |
InChI |
InChI=1S/C13H19N5O5/c1-6(20)4-22-12-10-11(16-13(14)17-12)18(5-15-10)9-2-7(21)8(3-19)23-9/h5-9,19-21H,2-4H2,1H3,(H2,14,16,17)/t6?,7-,8-,9-/m1/s1 | |
Source | PubChem | |
URL | https://pubchem.ncbi.nlm.nih.gov | |
Description | Data deposited in or computed by PubChem | |
InChI Key |
HLFWWFZDUMLOEE-FUZJYRNYSA-N | |
Source | PubChem | |
URL | https://pubchem.ncbi.nlm.nih.gov | |
Description | Data deposited in or computed by PubChem | |
Canonical SMILES |
CC(COC1=NC(=NC2=C1N=CN2C3CC(C(O3)CO)O)N)O | |
Source | PubChem | |
URL | https://pubchem.ncbi.nlm.nih.gov | |
Description | Data deposited in or computed by PubChem | |
Isomeric SMILES |
CC(COC1=NC(=NC2=C1N=CN2[C@H]3C[C@H]([C@H](O3)CO)O)N)O | |
Source | PubChem | |
URL | https://pubchem.ncbi.nlm.nih.gov | |
Description | Data deposited in or computed by PubChem | |
Molecular Formula |
C13H19N5O5 | |
Source | PubChem | |
URL | https://pubchem.ncbi.nlm.nih.gov | |
Description | Data deposited in or computed by PubChem | |
DSSTOX Substance ID |
DTXSID60857835 | |
Record name | 9-(2-Deoxy-beta-D-threo-pentofuranosyl)-6-(2-hydroxypropoxy)-9H-purin-2-amine | |
Source | EPA DSSTox | |
URL | https://comptox.epa.gov/dashboard/DTXSID60857835 | |
Description | DSSTox provides a high quality public chemistry resource for supporting improved predictive toxicology. | |
Molecular Weight |
325.32 g/mol | |
Source | PubChem | |
URL | https://pubchem.ncbi.nlm.nih.gov | |
Description | Data deposited in or computed by PubChem | |
CAS No. |
1327339-22-9 | |
Record name | 9-(2-Deoxy-beta-D-threo-pentofuranosyl)-6-(2-hydroxypropoxy)-9H-purin-2-amine | |
Source | EPA DSSTox | |
URL | https://comptox.epa.gov/dashboard/DTXSID60857835 | |
Description | DSSTox provides a high quality public chemistry resource for supporting improved predictive toxicology. | |
Disclaimer and Information on In-Vitro Research Products
Please be aware that all articles and product information presented on BenchChem are intended solely for informational purposes. The products available for purchase on BenchChem are specifically designed for in-vitro studies, which are conducted outside of living organisms. In-vitro studies, derived from the Latin term "in glass," involve experiments performed in controlled laboratory settings using cells or tissues. It is important to note that these products are not categorized as medicines or drugs, and they have not received approval from the FDA for the prevention, treatment, or cure of any medical condition, ailment, or disease. We must emphasize that any form of bodily introduction of these products into humans or animals is strictly prohibited by law. It is essential to adhere to these guidelines to ensure compliance with legal and ethical standards in research and experimentation.