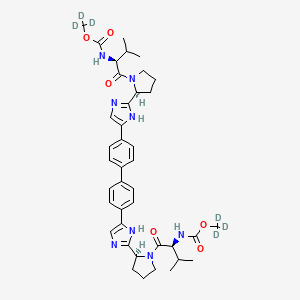
Melphalan Dimer-d8 Dihydrochloride
Overview
Description
Daclatasvir-d6 is a deuterium-labeled derivative of Daclatasvir, a potent and orally active inhibitor of the hepatitis C virus (HCV) non-structural protein 5A (NS5A). This compound is primarily used as an internal standard in mass spectrometry for the quantification of Daclatasvir. The deuterium labeling helps in distinguishing it from the non-labeled compound during analytical procedures .
Scientific Research Applications
Daclatasvir-d6 has a wide range of scientific research applications:
Chemistry: Used as an internal standard in mass spectrometry for the quantification of Daclatasvir and its metabolites.
Biology: Helps in studying the pharmacokinetics and metabolism of Daclatasvir in biological systems.
Medicine: Assists in the development and optimization of antiviral therapies targeting HCV.
Industry: Employed in quality control and analytical testing of pharmaceutical formulations containing Daclatasvir
Safety and Hazards
Melphalan is considered hazardous by the 2012 OSHA Hazard Communication Standard . It is fatal if swallowed, in contact with skin, or if inhaled . It may cause cancer and is suspected of damaging fertility or the unborn child . It is advised to obtain special instructions before use and not to handle until all safety precautions have been read and understood .
Mechanism of Action
Target of Action
Melphalan Dimer-d8 Dihydrochloride, also known as Melphalan, is an alkylating agent of the bischloroethylamine type . It primarily targets DNA within tumor cells . It is believed to be taken up by tumor cells via a neutral amino acid active pathway shared by leucine .
Mode of Action
Melphalan binds at the N7 position of guanine and induces inter-strand cross-links in DNA . This disrupts DNA synthesis or transcription, thereby inhibiting the proliferation of tumor cells .
Biochemical Pathways
The primary biochemical pathway affected by Melphalan is DNA replication. By inducing inter-strand cross-links in DNA, Melphalan disrupts the normal replication process. This leads to cell cycle arrest and ultimately cell death .
Pharmacokinetics
Melphalan, the parent compound, is known to have a terminal elimination half-life of approximately 75 minutes following intravenous administration .
Result of Action
The primary result of Melphalan’s action is the inhibition of DNA replication, leading to cell cycle arrest and cell death . This makes it effective in the treatment of various cancers, including multiple myeloma and ovarian carcinoma .
Biochemical Analysis
Biochemical Properties
Melphalan Dimer-d8 Dihydrochloride: plays a significant role in biochemical reactions by forming covalent bonds with DNA, leading to cross-linking and subsequent disruption of DNA replication and transcription. This compound interacts with various enzymes, proteins, and other biomolecules. For instance, it is known to interact with DNA polymerase and topoisomerase, enzymes crucial for DNA replication and repair. The nature of these interactions involves the formation of covalent bonds between the alkylating groups of this compound and the nucleophilic sites on the DNA and proteins, leading to the inhibition of their normal functions .
Cellular Effects
This compound: exerts profound effects on various types of cells and cellular processes. It influences cell function by inducing DNA damage, which triggers cell cycle arrest and apoptosis. This compound affects cell signaling pathways, such as the p53 pathway, which is activated in response to DNA damage and leads to the expression of genes involved in cell cycle regulation and apoptosis. Additionally, this compound impacts cellular metabolism by disrupting the normal function of enzymes involved in metabolic pathways, leading to altered metabolite levels and metabolic flux .
Molecular Mechanism
The molecular mechanism of This compound involves its ability to form covalent bonds with DNA and proteins, leading to the inhibition of DNA replication and transcription. This compound binds to the nucleophilic sites on DNA, such as the N7 position of guanine, resulting in the formation of DNA adducts and cross-links. These modifications prevent the normal unwinding and separation of DNA strands, thereby inhibiting the activity of DNA polymerase and topoisomerase. Additionally, this compound can induce changes in gene expression by activating DNA damage response pathways, leading to the upregulation of genes involved in DNA repair and apoptosis .
Temporal Effects in Laboratory Settings
In laboratory settings, the effects of This compound change over time. The compound’s stability and degradation are critical factors that influence its long-term effects on cellular function. Studies have shown that this compound is relatively stable under controlled conditions, but it can degrade over time, leading to a decrease in its efficacy. Long-term exposure to this compound in in vitro or in vivo studies has been associated with sustained DNA damage, prolonged cell cycle arrest, and increased apoptosis .
Dosage Effects in Animal Models
The effects of This compound vary with different dosages in animal models. At low doses, the compound can induce DNA damage and cell cycle arrest without causing significant toxicity. At higher doses, this compound can lead to severe toxic effects, including bone marrow suppression, gastrointestinal toxicity, and systemic organ damage. Threshold effects have been observed, where a certain dosage level is required to achieve a therapeutic effect, beyond which the adverse effects outweigh the benefits .
Metabolic Pathways
This compound: is involved in several metabolic pathways, primarily related to its alkylating activity. The compound interacts with enzymes such as glutathione S-transferase, which plays a role in detoxifying alkylating agents. This interaction leads to the formation of conjugates that are more easily excreted from the body. Additionally, this compound can affect metabolic flux by altering the activity of enzymes involved in glycolysis and the tricarboxylic acid cycle, leading to changes in metabolite levels .
Transport and Distribution
The transport and distribution of This compound within cells and tissues are mediated by various transporters and binding proteins. The compound is taken up by cells through passive diffusion and active transport mechanisms. Once inside the cell, it can bind to intracellular proteins and DNA, leading to its accumulation in specific cellular compartments. The distribution of this compound within tissues is influenced by factors such as blood flow, tissue permeability, and the presence of binding proteins .
Subcellular Localization
This compound: exhibits specific subcellular localization, which affects its activity and function. The compound is primarily localized in the nucleus, where it interacts with DNA and nuclear proteins. This localization is facilitated by the presence of targeting signals and post-translational modifications that direct this compound to the nucleus. The nuclear localization of this compound is crucial for its ability to induce DNA damage and inhibit DNA replication and transcription .
Preparation Methods
Synthetic Routes and Reaction Conditions
The synthesis of Daclatasvir-d6 involves the incorporation of deuterium atoms into the Daclatasvir molecule. This is typically achieved through hydrogen-deuterium exchange reactions or by using deuterated reagents during the synthesis process. The key steps include:
Hydrogen-Deuterium Exchange: This method involves replacing hydrogen atoms in the Daclatasvir molecule with deuterium atoms using deuterium gas or deuterated solvents under specific conditions.
Deuterated Reagents: Using deuterated starting materials or intermediates in the synthesis of Daclatasvir ensures the incorporation of deuterium atoms at desired positions in the final product.
Industrial Production Methods
Industrial production of Daclatasvir-d6 follows similar synthetic routes but on a larger scale. The process involves:
Bulk Synthesis: Large-scale hydrogen-deuterium exchange reactions or the use of deuterated reagents.
Purification: The product is purified using techniques such as crystallization, chromatography, or distillation to achieve high purity levels.
Quality Control: Rigorous testing using mass spectrometry and other analytical methods to ensure the correct incorporation of deuterium and the purity of the final product.
Chemical Reactions Analysis
Types of Reactions
Daclatasvir-d6 undergoes various chemical reactions, including:
Oxidation: The compound can be oxidized under specific conditions to form oxidized derivatives.
Reduction: Reduction reactions can convert certain functional groups within the molecule to their reduced forms.
Substitution: Substitution reactions can occur, where specific atoms or groups in the molecule are replaced by other atoms or groups.
Common Reagents and Conditions
Oxidation: Common oxidizing agents include potassium permanganate, chromium trioxide, and hydrogen peroxide.
Reduction: Reducing agents such as lithium aluminum hydride, sodium borohydride, and hydrogen gas are used.
Substitution: Reagents like halogens, acids, and bases are employed in substitution reactions.
Major Products Formed
The major products formed from these reactions depend on the specific conditions and reagents used. For example, oxidation may yield hydroxylated or ketone derivatives, while reduction may produce alcohols or amines .
Comparison with Similar Compounds
Similar Compounds
Ledipasvir: Another NS5A inhibitor used in combination with other antiviral agents for the treatment of HCV.
Ombitasvir: An NS5A inhibitor used in combination therapies for HCV.
Elbasvir: Similar to Daclatasvir, it inhibits the NS5A protein of HCV.
Uniqueness of Daclatasvir-d6
Daclatasvir-d6 is unique due to its deuterium labeling, which provides distinct advantages in analytical applications. The deuterium atoms make it easily distinguishable from non-labeled Daclatasvir in mass spectrometry, enhancing the accuracy and reliability of quantitative analyses .
Properties
IUPAC Name |
trideuteriomethyl N-[(2S)-3-methyl-1-[(2S)-2-[5-[4-[4-[2-[(2S)-1-[(2S)-3-methyl-2-(trideuteriomethoxycarbonylamino)butanoyl]pyrrolidin-2-yl]-1H-imidazol-5-yl]phenyl]phenyl]-1H-imidazol-2-yl]pyrrolidin-1-yl]-1-oxobutan-2-yl]carbamate | |
---|---|---|
Source | PubChem | |
URL | https://pubchem.ncbi.nlm.nih.gov | |
Description | Data deposited in or computed by PubChem | |
InChI |
InChI=1S/C40H50N8O6/c1-23(2)33(45-39(51)53-5)37(49)47-19-7-9-31(47)35-41-21-29(43-35)27-15-11-25(12-16-27)26-13-17-28(18-14-26)30-22-42-36(44-30)32-10-8-20-48(32)38(50)34(24(3)4)46-40(52)54-6/h11-18,21-24,31-34H,7-10,19-20H2,1-6H3,(H,41,43)(H,42,44)(H,45,51)(H,46,52)/t31-,32-,33-,34-/m0/s1/i5D3,6D3 | |
Source | PubChem | |
URL | https://pubchem.ncbi.nlm.nih.gov | |
Description | Data deposited in or computed by PubChem | |
InChI Key |
FKRSSPOQAMALKA-GXBFPSLASA-N | |
Source | PubChem | |
URL | https://pubchem.ncbi.nlm.nih.gov | |
Description | Data deposited in or computed by PubChem | |
Canonical SMILES |
CC(C)C(C(=O)N1CCCC1C2=NC=C(N2)C3=CC=C(C=C3)C4=CC=C(C=C4)C5=CN=C(N5)C6CCCN6C(=O)C(C(C)C)NC(=O)OC)NC(=O)OC | |
Source | PubChem | |
URL | https://pubchem.ncbi.nlm.nih.gov | |
Description | Data deposited in or computed by PubChem | |
Isomeric SMILES |
[2H]C([2H])([2H])OC(=O)N[C@@H](C(C)C)C(=O)N1CCC[C@H]1C2=NC=C(N2)C3=CC=C(C=C3)C4=CC=C(C=C4)C5=CN=C(N5)[C@@H]6CCCN6C(=O)[C@H](C(C)C)NC(=O)OC([2H])([2H])[2H] | |
Source | PubChem | |
URL | https://pubchem.ncbi.nlm.nih.gov | |
Description | Data deposited in or computed by PubChem | |
Molecular Formula |
C40H50N8O6 | |
Source | PubChem | |
URL | https://pubchem.ncbi.nlm.nih.gov | |
Description | Data deposited in or computed by PubChem | |
Molecular Weight |
744.9 g/mol | |
Source | PubChem | |
URL | https://pubchem.ncbi.nlm.nih.gov | |
Description | Data deposited in or computed by PubChem | |
Retrosynthesis Analysis
AI-Powered Synthesis Planning: Our tool employs the Template_relevance Pistachio, Template_relevance Bkms_metabolic, Template_relevance Pistachio_ringbreaker, Template_relevance Reaxys, Template_relevance Reaxys_biocatalysis model, leveraging a vast database of chemical reactions to predict feasible synthetic routes.
One-Step Synthesis Focus: Specifically designed for one-step synthesis, it provides concise and direct routes for your target compounds, streamlining the synthesis process.
Accurate Predictions: Utilizing the extensive PISTACHIO, BKMS_METABOLIC, PISTACHIO_RINGBREAKER, REAXYS, REAXYS_BIOCATALYSIS database, our tool offers high-accuracy predictions, reflecting the latest in chemical research and data.
Strategy Settings
Precursor scoring | Relevance Heuristic |
---|---|
Min. plausibility | 0.01 |
Model | Template_relevance |
Template Set | Pistachio/Bkms_metabolic/Pistachio_ringbreaker/Reaxys/Reaxys_biocatalysis |
Top-N result to add to graph | 6 |
Feasible Synthetic Routes
Disclaimer and Information on In-Vitro Research Products
Please be aware that all articles and product information presented on BenchChem are intended solely for informational purposes. The products available for purchase on BenchChem are specifically designed for in-vitro studies, which are conducted outside of living organisms. In-vitro studies, derived from the Latin term "in glass," involve experiments performed in controlled laboratory settings using cells or tissues. It is important to note that these products are not categorized as medicines or drugs, and they have not received approval from the FDA for the prevention, treatment, or cure of any medical condition, ailment, or disease. We must emphasize that any form of bodily introduction of these products into humans or animals is strictly prohibited by law. It is essential to adhere to these guidelines to ensure compliance with legal and ethical standards in research and experimentation.