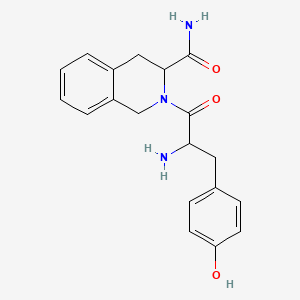
Tyr-Tic-NH2
- Click on QUICK INQUIRY to receive a quote from our team of experts.
- With the quality product at a COMPETITIVE price, you can focus more on your research.
Overview
Description
Tyr-Tic-NH2: is a synthetic peptide compound that consists of tyrosine (Tyr), 1,2,3,4-tetrahydroisoquinoline-3-carboxylic acid (Tic), and an amide group (NH2). This compound is notable for its role as a delta opioid receptor antagonist, which means it can block the action of certain opioid peptides that bind to delta opioid receptors .
Preparation Methods
Synthetic Routes and Reaction Conditions: The synthesis of Tyr-Tic-NH2 typically involves solid-phase peptide synthesis (SPPS) techniques. The process begins with the attachment of the first amino acid, tyrosine, to a solid resin. Subsequent amino acids, including 1,2,3,4-tetrahydroisoquinoline-3-carboxylic acid, are added sequentially using Fmoc (9-fluorenylmethyloxycarbonyl) chemistry. The final step involves the cleavage of the peptide from the resin and the addition of the amide group (NH2) to the C-terminus .
Industrial Production Methods: Industrial production of this compound follows similar synthetic routes but on a larger scale. Automated peptide synthesizers are often employed to ensure precision and efficiency. The purification of the final product is typically achieved through high-performance liquid chromatography (HPLC) to ensure high purity .
Chemical Reactions Analysis
Types of Reactions: Tyr-Tic-NH2 can undergo various chemical reactions, including:
Oxidation: The tyrosine residue can be oxidized to form dityrosine or other oxidative products.
Reduction: Reduction reactions can target the amide bond, although this is less common.
Substitution: The aromatic ring of tyrosine can undergo electrophilic substitution reactions.
Common Reagents and Conditions:
Oxidation: Hydrogen peroxide or other oxidizing agents.
Reduction: Sodium borohydride or other reducing agents.
Substitution: Halogenating agents or nitrating agents for electrophilic substitution.
Major Products:
Oxidation: Dityrosine or other oxidized derivatives.
Reduction: Reduced amide derivatives.
Substitution: Halogenated or nitrated tyrosine derivatives.
Scientific Research Applications
Tyr-Tic-NH2 has a wide range of applications in scientific research:
Chemistry: Used as a model compound to study peptide synthesis and modifications.
Biology: Investigated for its role in modulating opioid receptors and its potential effects on pain perception.
Medicine: Explored as a potential therapeutic agent for conditions involving opioid receptors, such as pain management and addiction treatment.
Industry: Utilized in the development of new peptide-based drugs and as a reference compound in analytical techniques
Mechanism of Action
Tyr-Tic-NH2 exerts its effects by binding to delta opioid receptors and blocking the action of endogenous opioid peptides. This antagonistic action prevents the activation of the receptor, thereby inhibiting the downstream signaling pathways that would normally be triggered by opioid binding. The molecular targets include the delta opioid receptors, and the pathways involved are primarily related to pain modulation and neurotransmitter release .
Comparison with Similar Compounds
Tyr-Tic-Ala: Another delta opioid receptor antagonist that lacks the phenylalanine residue.
H-Dmt-Tic-OH: A highly selective delta opioid receptor antagonist with increased affinity and selectivity compared to Tyr-Tic-NH2.
H-Dmt-Tic-NH2: Similar to H-Dmt-Tic-OH but with an amide group, showing different receptor binding profiles
Uniqueness: this compound is unique due to its specific structure, which allows it to selectively bind to delta opioid receptors without the presence of phenylalanine, a residue typically considered essential for opioid activity. This makes it a valuable tool in studying the structure-activity relationships of opioid peptides and developing new therapeutic agents .
Properties
IUPAC Name |
(3S)-2-[(2S)-2-amino-3-(4-hydroxyphenyl)propanoyl]-3,4-dihydro-1H-isoquinoline-3-carboxamide |
Source
|
---|---|---|
Source | PubChem | |
URL | https://pubchem.ncbi.nlm.nih.gov | |
Description | Data deposited in or computed by PubChem | |
InChI |
InChI=1S/C19H21N3O3/c20-16(9-12-5-7-15(23)8-6-12)19(25)22-11-14-4-2-1-3-13(14)10-17(22)18(21)24/h1-8,16-17,23H,9-11,20H2,(H2,21,24)/t16-,17-/m0/s1 |
Source
|
Source | PubChem | |
URL | https://pubchem.ncbi.nlm.nih.gov | |
Description | Data deposited in or computed by PubChem | |
InChI Key |
KIHQBNDANGJYBJ-IRXDYDNUSA-N |
Source
|
Source | PubChem | |
URL | https://pubchem.ncbi.nlm.nih.gov | |
Description | Data deposited in or computed by PubChem | |
Canonical SMILES |
C1C(N(CC2=CC=CC=C21)C(=O)C(CC3=CC=C(C=C3)O)N)C(=O)N |
Source
|
Source | PubChem | |
URL | https://pubchem.ncbi.nlm.nih.gov | |
Description | Data deposited in or computed by PubChem | |
Isomeric SMILES |
C1[C@H](N(CC2=CC=CC=C21)C(=O)[C@H](CC3=CC=C(C=C3)O)N)C(=O)N |
Source
|
Source | PubChem | |
URL | https://pubchem.ncbi.nlm.nih.gov | |
Description | Data deposited in or computed by PubChem | |
Molecular Formula |
C19H21N3O3 |
Source
|
Source | PubChem | |
URL | https://pubchem.ncbi.nlm.nih.gov | |
Description | Data deposited in or computed by PubChem | |
Molecular Weight |
339.4 g/mol |
Source
|
Source | PubChem | |
URL | https://pubchem.ncbi.nlm.nih.gov | |
Description | Data deposited in or computed by PubChem | |
Disclaimer and Information on In-Vitro Research Products
Please be aware that all articles and product information presented on BenchChem are intended solely for informational purposes. The products available for purchase on BenchChem are specifically designed for in-vitro studies, which are conducted outside of living organisms. In-vitro studies, derived from the Latin term "in glass," involve experiments performed in controlled laboratory settings using cells or tissues. It is important to note that these products are not categorized as medicines or drugs, and they have not received approval from the FDA for the prevention, treatment, or cure of any medical condition, ailment, or disease. We must emphasize that any form of bodily introduction of these products into humans or animals is strictly prohibited by law. It is essential to adhere to these guidelines to ensure compliance with legal and ethical standards in research and experimentation.