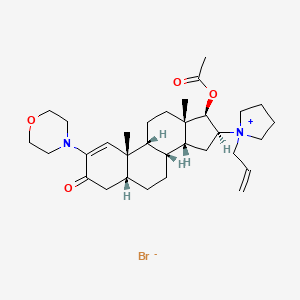
Rocuronium Bromide EP Impurity H Bromide
- Click on QUICK INQUIRY to receive a quote from our team of experts.
- With the quality product at a COMPETITIVE price, you can focus more on your research.
Overview
Description
Rocuronium bromide is a neuromuscular blocking agent used in the medical field to produce muscle relaxation during anesthesia and surgery. It is a synthetic, non-depolarizing neuromuscular blocker, which is structurally related to vecuronium bromide. EP Impurity H Bromide is a metabolite of rocuronium bromide and its presence in the drug has been identified as a potential impurity.
Scientific Research Applications
Synthesis and Analytical Methodologies
- Rocuronium bromide, known for its use as a non-depolarizing neuromuscular blocker and muscle relaxant, has been the focus of research aiming to develop new and more efficient synthesis methods. One such study introduced an efficient route for preparing a key intermediate, which could potentially enhance the synthesis of rocuronium bromide while reducing the generation of difficult-to-remove impurities (Wu et al., 2017).
- Analytical methods have been established for the determination of rocuronium bromide and its related substances, including impurities. HPLC techniques using various columns and mobile phases have been developed to achieve separation and quantification of rocuronium bromide and its impurities, thereby ensuring the quality of pharmaceutical preparations (Kai, 2010); (Błażewicz et al., 2007).
Degradation and Stability Studies
- Degradation behavior under various stress conditions has been studied to understand the stability of rocuronium bromide. Such studies are crucial for the development of stable pharmaceutical formulations and for understanding the chemical behavior of the drug under different conditions (Wegener et al., 2015); (El Houssini et al., 2021).
Reversal Agents for Rocuronium Bromide
- Research into reversal agents for rocuronium bromide has been conducted to find compounds that can safely and effectively counteract the muscle relaxation effects of rocuronium bromide. This is particularly important in the context of anesthesia, where rapid recovery from muscle relaxation is often required. Cyclodextrin derivatives have been explored as potential reversal agents due to their ability to form host-guest complexes with rocuronium, providing a novel approach to reversing neuromuscular blockage without the cardiovascular side effects associated with traditional methods (Adam et al., 2002).
Mechanism of Action
Target of Action
1,2-Dehydro-3-oxo Rocuronium Bromide, also known as Rocuronium Bromide EP Impurity H Bromide, is an impurity of Rocuronium Bromide . Rocuronium Bromide is an aminosteroid and a competitive neuromuscular blocker . Therefore, the primary target of 1,2-Dehydro-3-oxo Rocuronium Bromide is likely to be the neuromuscular junction, specifically the nicotinic acetylcholine receptors located there.
Mode of Action
As a competitive neuromuscular blocker, 1,2-Dehydro-3-oxo Rocuronium Bromide is expected to bind to the nicotinic acetylcholine receptors at the neuromuscular junction. This binding prevents the action of acetylcholine, a neurotransmitter responsible for muscle contraction, thereby causing muscle relaxation .
Biochemical Pathways
As an analogue of rocuronium bromide, it is expected to interfere with the normal function of the neuromuscular junction, preventing muscle contraction and causing muscle relaxation .
Pharmacokinetics
Rocuronium Bromide is typically administered intravenously, and its effects are rapid in onset .
Result of Action
The primary result of the action of 1,2-Dehydro-3-oxo Rocuronium Bromide is expected to be muscle relaxation, due to its role as a competitive neuromuscular blocker . This can facilitate procedures such as endotracheal intubation and provide muscle relaxation during surgery .
Action Environment
The action of 1,2-Dehydro-3-oxo Rocuronium Bromide, like other neuromuscular blockers, can be influenced by various environmental factors. For instance, its stability and efficacy may be affected by temperature and pH . .
Safety and Hazards
Biochemical Analysis
Biochemical Properties
Rocuronium Bromide EP Impurity H Bromide works by binding to the nicotinic receptor at the motor end plate, antagonizing acetylcholine binding, resulting in skeletal muscle relaxation and paralysis . This interaction with the nicotinic receptor, a type of protein, is crucial for its function .
Cellular Effects
The primary cellular effect of this compound is the induction of skeletal muscle relaxation. This is achieved by its antagonistic action on the nicotinic acetylcholine receptors located at the motor end plate . This interaction disrupts normal cellular signaling pathways, leading to muscle relaxation and paralysis .
Molecular Mechanism
The molecular mechanism of action of this compound involves binding to the nicotinic acetylcholine receptors at the neuromuscular junction . This binding antagonizes the action of acetylcholine, a neurotransmitter, thereby blocking the transmission of signals from the nerves to the muscles . This results in muscle relaxation and paralysis .
Temporal Effects in Laboratory Settings
It is known that the compound has a rapid onset and intermediate duration of action .
Metabolic Pathways
Rocuronium Bromide, the parent compound, is known to undergo some de-acetylation .
Subcellular Localization
The subcellular localization of this compound is likely to be at the cell membrane where the nicotinic acetylcholine receptors are located
properties
{ "Design of the Synthesis Pathway": "The synthesis pathway for Rocuronium Bromide EP Impurity H Bromide involves the conversion of Rocuronium Bromide to its corresponding impurity H, followed by bromination to obtain the final product.", "Starting Materials": [ "Rocuronium Bromide", "Bromine", "Sodium Bromide", "Acetic Acid", "Hydrogen Peroxide", "Water" ], "Reaction": [ "Step 1: Rocuronium Bromide is dissolved in acetic acid.", "Step 2: Hydrogen peroxide is added to the solution and the mixture is stirred at room temperature for 2 hours.", "Step 3: The reaction mixture is then poured into ice-cold water and the resulting precipitate is filtered and dried to obtain Rocuronium Bromide EP Impurity H.", "Step 4: Impurity H is dissolved in acetic acid and bromine is added dropwise with stirring.", "Step 5: Sodium bromide is added to the reaction mixture and the stirring is continued for 2 hours.", "Step 6: The reaction mixture is poured into ice-cold water and the resulting precipitate is filtered and dried to obtain Rocuronium Bromide EP Impurity H Bromide." ] } | |
CAS RN |
1190105-67-9 |
Molecular Formula |
C32H49BrN2O4 |
Molecular Weight |
605.6 g/mol |
IUPAC Name |
[10,13-dimethyl-2-morpholin-4-yl-3-oxo-16-(1-prop-2-enylpyrrolidin-1-ium-1-yl)-4,5,6,7,8,9,11,12,14,15,16,17-dodecahydrocyclopenta[a]phenanthren-17-yl] acetate;bromide |
InChI |
InChI=1S/C32H49N2O4.BrH/c1-5-14-34(15-6-7-16-34)28-20-26-24-9-8-23-19-29(36)27(33-12-17-37-18-13-33)21-32(23,4)25(24)10-11-31(26,3)30(28)38-22(2)35;/h5,21,23-26,28,30H,1,6-20H2,2-4H3;1H/q+1;/p-1 |
InChI Key |
FDZFJGLEXRXQBW-UHFFFAOYSA-M |
Isomeric SMILES |
CC(=O)O[C@H]1[C@H](C[C@@H]2[C@@]1(CC[C@H]3[C@H]2CC[C@@H]4[C@@]3(C=C(C(=O)C4)N5CCOCC5)C)C)[N+]6(CCCC6)CC=C.[Br-] |
SMILES |
CC(=O)OC1C(CC2C1(CCC3C2CCC4C3(C=C(C(=O)C4)N5CCOCC5)C)C)[N+]6(CCCC6)CC=C.[Br-] |
Canonical SMILES |
CC(=O)OC1C(CC2C1(CCC3C2CCC4C3(C=C(C(=O)C4)N5CCOCC5)C)C)[N+]6(CCCC6)CC=C.[Br-] |
synonyms |
1-[(5α,16β,17β)-17-(Acetyloxy)-2-(4-morpholinyl)-3-oxoandrost-1-en-16-yl]-1-(2-propen-1-yl)-pyrrolidinium Bromide |
Origin of Product |
United States |
Disclaimer and Information on In-Vitro Research Products
Please be aware that all articles and product information presented on BenchChem are intended solely for informational purposes. The products available for purchase on BenchChem are specifically designed for in-vitro studies, which are conducted outside of living organisms. In-vitro studies, derived from the Latin term "in glass," involve experiments performed in controlled laboratory settings using cells or tissues. It is important to note that these products are not categorized as medicines or drugs, and they have not received approval from the FDA for the prevention, treatment, or cure of any medical condition, ailment, or disease. We must emphasize that any form of bodily introduction of these products into humans or animals is strictly prohibited by law. It is essential to adhere to these guidelines to ensure compliance with legal and ethical standards in research and experimentation.