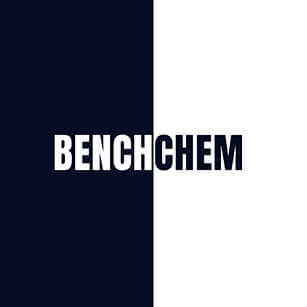
Gemcitabine Diphosphate Choline
- Click on QUICK INQUIRY to receive a quote from our team of experts.
- With the quality product at a COMPETITIVE price, you can focus more on your research.
Overview
Description
Gemcitabine diphosphate choline is a significant metabolite of gemcitabine, a nucleoside analog used in chemotherapy. This compound is linked to the Kennedy pathway, which is crucial in the metabolism of phospholipids in cells. This compound has been studied extensively in the context of pancreatic ductal adenocarcinoma, where it plays a role in the drug’s metabolic pathway .
Mechanism of Action
Target of Action
Gemcitabine Diphosphate Choline primarily targets DNA synthesis and ribonucleotide reductase . Ribonucleotide reductase is an enzyme that catalyzes the formation of deoxyribonucleotide triphosphates required for DNA synthesis .
Mode of Action
Gemcitabine, a cytidine analog, is transformed into its active metabolites, gemcitabine diphosphate (dFdCDP) and gemcitabine triphosphate (dFdCTP), that work by replacing the building blocks of nucleic acids during DNA elongation . This results in the arrest of tumor growth and promotes apoptosis of malignant cells .
Biochemical Pathways
Gemcitabine undergoes complex intracellular conversion to the nucleotides gemcitabine diphosphate (dFdCDP) and triphosphate (dFdCTP), which are responsible for its cytotoxic actions . The cytotoxic activity of gemcitabine may be the result of several actions on DNA synthesis. dFdCTP competes with deoxycytidine triphosphate (dCTP) as an inhibitor of DNA polymerase. dFdCDP is a potent inhibitor of ribonucleotide reductase, resulting in depletion of deoxyribonucleotide pools necessary for DNA synthesis and, thereby potentiating the effects of dFdCTP .
Pharmacokinetics
Gemcitabine is a pro-drug and, once transported into the cell, must be phosphorylated by deoxycytidine kinase to an active form . Both gemcitabine diphosphate (dFdCTP) and gemcitabine triphosphate (dFdCTP) inhibit processes required for DNA synthesis . Gemcitabine can be effectively inactivated mainly by the action of deoxycytidine deaminase to 2,29-difluorodeoxyuridine .
Result of Action
The incorporation of dFdCTP into DNA is most likely the major mechanism by which gemcitabine causes cell death . It terminates DNA chain elongation, arresting tumor growth and promoting apoptosis of malignant cells .
Action Environment
The efficacy of gemcitabine can be influenced by various environmental factors. For instance, the presence of cytidine triphosphate (CTP) can inhibit the formation of this compound from dFdCTP, indicating competition between CTP and dFdCTP for CTP:phosphocholine cytidylyltransferase (CCT) . Additionally, the liver disposition of gemcitabine, which is responsible for the metabolism of gemcitabine, can also impact the drug’s efficacy .
Biochemical Analysis
Biochemical Properties
Gemcitabine Diphosphate Choline plays a crucial role in biochemical reactions. It is produced through the metabolic activation of Gemcitabine in cells, initiated by deoxycytidine kinase (dCK) to give the monophosphate . Further sequential phosphorylation yields the diphosphate and triphosphate, both responsible for the cytotoxic effects of the drug . The formation of this compound from dFdCTP indicates competition between CTP and dFdCTP for CTP:phosphocholine cytidylyltransferase (CCT) .
Cellular Effects
This compound has significant effects on various types of cells and cellular processes. It influences cell function by impacting cell signaling pathways, gene expression, and cellular metabolism . As the structure of this compound precludes entry into cells, potential cytotoxicity was assessed by stimulating CCT activity using linoleate in KPC cells in vitro, leading to increased this compound concentration and synergistic growth inhibition after Gemcitabine addition .
Molecular Mechanism
This compound exerts its effects at the molecular level through various mechanisms. It competes with deoxycytidine triphosphate (dCTP) as an inhibitor of DNA polymerase . Gemcitabine Diphosphate inhibits ribonucleotide reductase, which catalyses the formation of deoxynucleotide triphosphates required for DNA synthesis . When incorporated into DNA, Gemcitabine triphosphate terminates DNA chain elongation .
Metabolic Pathways
This compound is involved in the Kennedy pathway, a metabolic pathway that synthesizes phosphatidylcholine from choline . It interacts with enzymes such as deoxycytidine kinase and CTP:phosphocholine cytidylyltransferase .
Preparation Methods
Synthetic Routes and Reaction Conditions: The synthesis of gemcitabine diphosphate choline involves the metabolic activation of gemcitabine. Initially, gemcitabine is phosphorylated by deoxycytidine kinase to form gemcitabine monophosphate. This is followed by further phosphorylation to produce gemcitabine diphosphate and finally gemcitabine triphosphate. This compound is formed through the Kennedy pathway, where it competes with cytidine triphosphate for the enzyme CTP:phosphocholine cytidylyltransferase .
Industrial Production Methods: The focus is more on the production of gemcitabine itself, which is then metabolized in vivo to form this compound .
Chemical Reactions Analysis
Types of Reactions: Gemcitabine diphosphate choline undergoes several biochemical reactions, primarily phosphorylation and dephosphorylation. It is involved in the inhibition of ribonucleotide reductase, an enzyme crucial for DNA synthesis .
Common Reagents and Conditions: The formation of this compound involves enzymes such as deoxycytidine kinase and CTP:phosphocholine cytidylyltransferase. Conditions for these reactions typically involve physiological pH and temperature, as they occur within the cellular environment .
Major Products: The major products formed from the reactions involving this compound include gemcitabine triphosphate and cytidine triphosphate. These products play significant roles in the inhibition of DNA synthesis and the cytotoxic effects of gemcitabine .
Scientific Research Applications
Gemcitabine diphosphate choline has several scientific research applications, particularly in the study of pancreatic ductal adenocarcinoma. It is used to understand the metabolic pathways of gemcitabine and its effects on cancer cells. Research has shown that this compound is present in equimolar amounts to gemcitabine triphosphate in tumor tissues, indicating its importance in the drug’s mechanism of action .
Comparison with Similar Compounds
Similar Compounds:
- Gemcitabine triphosphate
- Cytidine triphosphate
- 20,20-difluorodeoxyuridine
Uniqueness: Gemcitabine diphosphate choline is unique in its role within the Kennedy pathway and its competition with cytidine triphosphate for enzymatic activity. Unlike other metabolites of gemcitabine, this compound is not incorporated into DNA but still plays a crucial role in the drug’s cytotoxic effects .
Properties
CAS No. |
1643126-46-8 |
---|---|
Molecular Formula |
C₁₄H₂₄F₂N₄O₁₀P₂ |
Molecular Weight |
508.31 |
Synonyms |
Gemcitabine 5’-Diphosphate Choline; |
Origin of Product |
United States |
Q1: What is the significance of Gemcitabine diphosphate choline as a metabolite of Gemcitabine in the context of pancreatic cancer?
A1: The research paper [] investigates the metabolic fate of Gemcitabine in pancreatic cancer models. The study identifies this compound as a major metabolite, suggesting its potential role in Gemcitabine's mechanism of action within these cancer cells. The formation of this metabolite is linked to the Kennedy pathway, a metabolic route responsible for the synthesis of phospholipids, crucial components of cell membranes. This finding suggests that this compound might influence pancreatic cancer cell behavior by interfering with lipid metabolism and membrane integrity.
Disclaimer and Information on In-Vitro Research Products
Please be aware that all articles and product information presented on BenchChem are intended solely for informational purposes. The products available for purchase on BenchChem are specifically designed for in-vitro studies, which are conducted outside of living organisms. In-vitro studies, derived from the Latin term "in glass," involve experiments performed in controlled laboratory settings using cells or tissues. It is important to note that these products are not categorized as medicines or drugs, and they have not received approval from the FDA for the prevention, treatment, or cure of any medical condition, ailment, or disease. We must emphasize that any form of bodily introduction of these products into humans or animals is strictly prohibited by law. It is essential to adhere to these guidelines to ensure compliance with legal and ethical standards in research and experimentation.