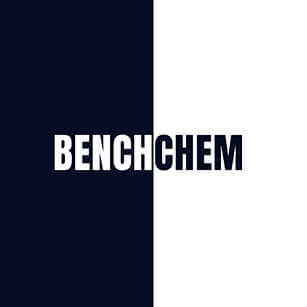
Taurocholic Acid-3-O-β-glucuronide Dipotassium Salt
- Click on QUICK INQUIRY to receive a quote from our team of experts.
- With the quality product at a COMPETITIVE price, you can focus more on your research.
Overview
Description
Taurocholic Acid-3-O-β-Glucuronide Dipotassium Salt (CAS: 75672-43-4) is a conjugated bile acid derivative. Structurally, it consists of taurine conjugated to cholic acid via an amide bond, with a β-glucuronide moiety at the 3-hydroxy position and two potassium counterions. This compound is primarily used in research as a reference standard for studying bile acid metabolism, drug interactions, and detoxification pathways . Its molecular formula is C₃₂H₄₉K₂NO₁₂S, with a molecular weight of 752.01 g/mol .
Preparation Methods
Synthetic Routes and Reaction Conditions
The synthesis of Taurocholic Acid-3-O-β-glucuronide Dipotassium Salt involves the glucuronidation of taurocholic acid. This process typically requires the use of glucuronic acid or its derivatives under specific reaction conditions to form the glucuronide conjugate. The reaction is usually catalyzed by enzymes such as UDP-glucuronosyltransferases, which facilitate the transfer of glucuronic acid to the hydroxyl group of taurocholic acid.
Industrial Production Methods
Industrial production of this compound involves large-scale glucuronidation processes. These processes are optimized for high yield and purity, often using bioreactors and advanced purification techniques to isolate the desired product.
Chemical Reactions Analysis
Types of Reactions
Taurocholic Acid-3-O-β-glucuronide Dipotassium Salt can undergo various chemical reactions, including:
Oxidation: This reaction involves the addition of oxygen or the removal of hydrogen, often using oxidizing agents.
Reduction: This reaction involves the addition of hydrogen or the removal of oxygen, typically using reducing agents.
Substitution: This reaction involves the replacement of one functional group with another, often facilitated by specific reagents and conditions.
Common Reagents and Conditions
Oxidation: Common oxidizing agents include potassium permanganate and hydrogen peroxide.
Reduction: Common reducing agents include sodium borohydride and lithium aluminum hydride.
Substitution: Common reagents include halogens and nucleophiles under appropriate conditions.
Major Products Formed
The major products formed from these reactions depend on the specific reagents and conditions used. For example, oxidation may yield hydroxylated derivatives, while reduction may produce dehydroxylated compounds.
Scientific Research Applications
Bile Acid Transport Studies
Taurocholic Acid-3-O-β-glucuronide Dipotassium Salt is utilized in research focusing on bile acid transport mechanisms. It serves as a substrate for studying the interaction of bile acids with transport proteins such as the Na+-dependent taurocholate cotransporting polypeptide (NTCP). For instance, studies have demonstrated its role in understanding how fluvastatin interacts with NTCP, which is crucial for drug development and understanding hepatic uptake of bile acids .
Metabolomics and Toxicology
This compound is significant in metabolomics studies, particularly those investigating hepatotoxicity. Research has shown that taurocholic acid levels can serve as biomarkers for liver diseases, including cirrhosis. A study involving human subjects indicated that elevated levels of taurocholic acid correlate with the progression of liver cirrhosis, suggesting its potential use as a therapeutic target .
Cholestatic Liver Disease Research
In studies examining cholestatic liver diseases, taurocholic acid has been shown to exert cholestatic effects through specific signaling pathways. For example, it has been implicated in phosphatidylinositol 3-kinase-dependent mechanisms that affect bile flow and liver function .
Drug Development
The compound's role as a cholagogue and cholerectic makes it valuable in drug formulations aimed at improving fat absorption and treating conditions related to bile acid deficiencies. Its interactions with various pharmacological agents are being explored to enhance therapeutic efficacy in gastrointestinal disorders .
Data Tables
Case Study 1: Hepatotoxicity Assessment
A study was conducted using rat models to assess the hepatotoxic effects of various compounds, including taurocholic acid derivatives. The results indicated significant alterations in bile acid profiles associated with liver damage markers, reinforcing the importance of taurocholic acid in assessing liver health .
Case Study 2: Bile Acid Profiling
In another investigation, urinary bile acids were profiled in piglets using targeted mass spectrometry techniques. The study highlighted the metabolic pathways involving taurocholic acid and its derivatives, providing insights into their roles in digestive health and disease states .
Mechanism of Action
The mechanism of action of Taurocholic Acid-3-O-β-glucuronide Dipotassium Salt involves its interaction with bile acid receptors and transporters. It modulates the absorption and metabolism of lipids by acting as a detergent to solubilize fats for absorption . The compound also interacts with specific molecular targets, such as the ileal sodium/bile acid cotransporter and G-protein coupled bile acid receptor 1, influencing various metabolic pathways .
Comparison with Similar Compounds
Structural and Functional Group Variations
Taurochenodeoxycholic Acid-3-O-β-Glucuronide Dipotassium Salt (CAS: 75672-23-0)
- Structural Difference: Replaces cholic acid with chenodeoxycholic acid (lacks a 12α-hydroxyl group).
- Molecular Formula: C₃₂H₄₉K₂NO₁₂S.
- Application : Used to study the enterohepatic circulation of bile acids and their role in cholesterol homeostasis .
12-Hydroxy Taurolithocholic Acid O-3-Glucuronide Dipotassium Salt (CAS: 75672-37-6)
- Structural Difference : Contains a lithocholic acid backbone (lacks 7α- and 12α-hydroxyl groups) with an additional 12-hydroxy modification.
- Molecular Weight : 752.01 g/mol.
- Role : Acts as an impurity of cholic acid, highlighting the importance of hydroxyl group positioning in bile acid solubility and toxicity .
Glycocholic Acid-3-O-β-Glucuronide Disodium Salt (CAS: 75672-42-3)
- Structural Difference : Substitutes taurine with glycine and uses disodium instead of dipotassium.
- Molecular Weight : 653.77 g/mol.
- Application : Investigated for its role in lipid digestion and as a biomarker for metabolic disorders .
Physicochemical Properties
Compound Name | CAS Number | Molecular Formula | Molecular Weight (g/mol) | Counterion | Solubility |
---|---|---|---|---|---|
Taurocholic Acid-3-O-β-Glucuronide Dipotassium Salt | 75672-43-4 | C₃₂H₄₉K₂NO₁₂S | 752.01 | Dipotassium | Data limited |
Taurochenodeoxycholic Acid-3-O-β-Glucuronide Dipotassium Salt | 75672-23-0 | C₃₂H₄₉K₂NO₁₂S | 752.01 | Dipotassium | Water-soluble |
Glycodeoxycholic Acid-3-O-β-Glucuronide Disodium Salt | 75672-36-5 | C₃₂H₄₉NNa₂O₁₁ | 669.71 | Disodium | Moderate in water |
Taurolithocholic Acid-3-O-Glucuronide Sulfate Disodium Salt | 75672-16-1 | C₃₂H₅₁NNa₂O₁₁S | 703.79 | Disodium | Hydrophilic |
Key Observations :
- Potassium salts (e.g., dipotassium) generally exhibit higher aqueous solubility than sodium salts due to smaller ionic radii and stronger hydration .
- Sulfated derivatives (e.g., ) show enhanced polarity, affecting membrane permeability and metabolic stability .
Stability and Handling
- Potassium vs. Sodium Salts : Dipotassium salts require stringent storage (-20°C) to prevent deliquescence, whereas disodium salts are more stable at room temperature .
- Sulfated Derivatives : Prone to hydrolysis under acidic conditions, necessitating pH-controlled environments .
Research Implications
Biological Activity
Taurocholic Acid-3-O-β-glucuronide Dipotassium Salt (TC-GUDPK) is a taurine-conjugated bile acid derivative that plays a significant role in bile acid metabolism and enterohepatic circulation. Understanding its biological activity is crucial for elucidating its potential therapeutic applications, particularly in liver function assessment and metabolic disorders.
TC-GUDPK is a dipotassium salt of taurocholic acid conjugated with glucuronic acid. Its structure enhances its solubility and bioavailability, making it an important compound in biochemical studies related to bile acids.
Property | Value |
---|---|
Molecular Formula | C₁₈H₃₃N₃O₇S |
Molecular Weight | 403.54 g/mol |
Solubility | Highly soluble in water |
1. Role in Bile Acid Metabolism
TC-GUDPK serves as a substrate for enzymes and transporters involved in bile acid biosynthesis and conjugation. It is synthesized in the liver and aids in the digestion and absorption of fats and fat-soluble vitamins by acting as a physiological detergent . Studies have shown that alterations in the levels of bile acids, including TC-GUDPK, can indicate liver dysfunction or disease .
2. Liver Function Assessment
Research indicates that TC-GUDPK can be utilized to assess liver function by evaluating its uptake and metabolism in hepatocytes. The liver's ability to synthesize and conjugate bile acids is critical for maintaining homeostasis; thus, studying TC-GUDPK can provide insights into hepatic health .
3. Detoxification Pathway
Sulfation of bile acids, including TC-GUDPK, is an important metabolic pathway for detoxification. This process increases the solubility of bile acids, facilitating their excretion via urine and feces. Notably, sulfation becomes more prominent during cholestatic conditions, where the body attempts to eliminate excess bile acids .
Case Study 1: Drug-Induced Liver Injury (DILI)
A study involving patients with drug-induced liver injury found significant correlations between serum levels of taurocholic acid (including TC-GUDPK) and the severity of liver damage. Elevated levels of taurocholic acid were associated with increased bilirubin levels and poorer outcomes in DILI patients .
Case Study 2: Bile Acid Transport Mechanisms
In Wistar rats with impaired biliary secretion due to genetic defects, the transport mechanisms for sulfated bile acids were significantly affected. The study highlighted how TC-GUDPK's metabolism could be altered under pathological conditions, suggesting its utility as a biomarker for assessing hepatic function .
Enzymatic Activity
Research has demonstrated that TC-GUDPK interacts with various enzymes involved in bile acid metabolism:
- Sulfotransferases : These enzymes catalyze the sulfation of bile acids, enhancing their solubility and excretion.
- Bile Salt Export Pump (BSEP) : Inhibition of BSEP has been linked to drug-induced liver injury; thus, understanding TC-GUDPK's interaction with this pump is vital for assessing drug safety profiles .
Microbiota Interaction
Recent studies have explored how gut microbiota can metabolize conjugated bile acids like TC-GUDPK into unconjugated forms through bacterial bile salt hydrolase activity. This transformation impacts the overall bile acid pool and can influence metabolic health .
Q & A
Basic Research Questions
Q. What are the standard methodologies for synthesizing and characterizing Taurocholic Acid-3-O-β-glucuronide Dipotassium Salt?
Synthesis typically involves enzymatic or chemical glucuronidation of taurocholic acid, followed by purification via high-performance liquid chromatography (HPLC) or solid-phase extraction. Characterization employs nuclear magnetic resonance (NMR) for structural confirmation and mass spectrometry (MS) for molecular weight verification. The CAS number (75672-43-4) is critical for compound identification in databases . Stability assessments should include pH-dependent degradation studies in aqueous solutions, monitored by UV-Vis spectroscopy or LC-MS .
Q. How should researchers prepare and standardize solutions of this compound for in vitro assays?
- Solution Preparation : Dissolve the compound in deionized water or phosphate-buffered saline (PBS) at neutral pH to avoid hydrolysis. For example, potassium-based test solutions (e.g., Potassium Hydroxide TS) require precise molarity adjustments to ensure solubility and stability .
- Dosage Calculations : Account for the dipotassium salt form versus the free acid. For instance, molecular weight adjustments are necessary when converting between salt and free acid concentrations (e.g., molecular weight 703.79 g/mol for the salt vs. 567.65 g/mol for the free acid) .
Q. What analytical techniques are recommended for quantifying this compound in biological matrices?
Liquid chromatography-tandem mass spectrometry (LC-MS/MS) is the gold standard due to high sensitivity and specificity. Use deuterated internal standards (e.g., taurocholic acid-d4) to correct for matrix effects. For tissue samples, homogenization followed by methanol extraction improves recovery rates .
Advanced Research Questions
Q. How can researchers investigate the role of this compound in bile acid transporters like SLC22A24?
- Experimental Design : Use HEK293 cells transfected with SLC22A24 to measure uptake kinetics. Competitive inhibition assays with steroid conjugates (e.g., etiocholanolone glucuronide) or bile acids (e.g., chenodeoxycholic acid) can identify substrate specificity. IC50 values for inhibitors (e.g., 1 mM dicarboxylic acids reduce uptake by ~50%) provide mechanistic insights .
- Data Interpretation : Compare uptake rates (e.g., [³H]-taurocholic acid at 1–2 minutes) between transfected and control cells to isolate transporter-specific activity .
Q. How should contradictions in therapeutic efficacy across disease models be addressed?
- Case Example : In ALS trials, TUDCA (a related bile acid) showed variable outcomes. Apply systematic review methods:
- Meta-Analysis : Use random-effects models to account for heterogeneity in study designs and species-specific metabolism .
Q. What strategies validate this compound as a biomarker in metabolic disorders like jaundice?
- Pathway Analysis : Map its role in "primary bile acid biosynthesis" via KEGG. Co-detection with bilirubin glucuronide in patient sera supports its utility as a core biomarker .
- Validation Cohorts : Use ROC analysis (AUC = 0.996 in jaundice vs. healthy subjects) to confirm diagnostic reliability. Independent cohorts (n=60) with unsupervised clustering (e.g., PCA) can verify reproducibility .
Q. Data Contradiction Analysis Example
Conflict : Discrepancies in toxicity profiles across studies.
Resolution :
- Compare experimental conditions (e.g., dipotassium salts of endothall showed low mussel mortality at 1–5 ppm but higher LC50 in controlled lab settings). Environmental factors (pH, temperature) may alter compound stability .
- Replicate studies using standardized protocols (e.g., OECD guidelines) to isolate confounding variables .
Properties
CAS No. |
75672-43-4 |
---|---|
Molecular Formula |
C₃₁H₄₉K₂NO₁₃S |
Molecular Weight |
753.98 |
Synonyms |
Cholane, β-D-Glucopyranosiduronic Acid Derivative; (3α,5β,7α,12α)-7,12-Dihydroxy-24-oxo-24-[(2-sulfoethyl)amino]cholan-3-yl, Dipotassium Salt |
Origin of Product |
United States |
Disclaimer and Information on In-Vitro Research Products
Please be aware that all articles and product information presented on BenchChem are intended solely for informational purposes. The products available for purchase on BenchChem are specifically designed for in-vitro studies, which are conducted outside of living organisms. In-vitro studies, derived from the Latin term "in glass," involve experiments performed in controlled laboratory settings using cells or tissues. It is important to note that these products are not categorized as medicines or drugs, and they have not received approval from the FDA for the prevention, treatment, or cure of any medical condition, ailment, or disease. We must emphasize that any form of bodily introduction of these products into humans or animals is strictly prohibited by law. It is essential to adhere to these guidelines to ensure compliance with legal and ethical standards in research and experimentation.