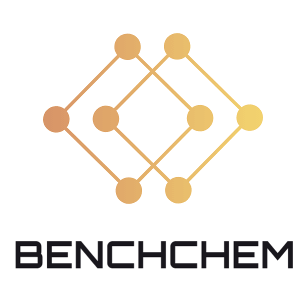
Cholic Acid 3-O-β-Glucuronide Disodium Salt
- Click on QUICK INQUIRY to receive a quote from our team of experts.
- With the quality product at a COMPETITIVE price, you can focus more on your research.
Description
Cholic Acid 3-O-β-Glucuronide Disodium Salt is a glycosidic-conjugated bile acid . It is a product of pharmaceutical toxicology and is used as a reference standard . It is also of research interest for its detection in urine .
Molecular Structure Analysis
The molecular formula of Cholic Acid 3-O-β-Glucuronide Disodium Salt is C30H46Na2O11 . The molecular weight is 628.7 g/mol . The structure includes a glucopyranosiduronic acid and a norcholic acid .Physical And Chemical Properties Analysis
The physical and chemical properties of Cholic Acid 3-O-β-Glucuronide Disodium Salt include a molecular weight of 628.7 g/mol, a hydrogen bond donor count of 5, a hydrogen bond acceptor count of 11, a rotatable bond count of 5, an exact mass of 628.28355084 g/mol, a monoisotopic mass of 628.28355084 g/mol, a topological polar surface area of 200 Ų, a heavy atom count of 43, and a formal charge of 0 .Scientific Research Applications
Dairy Production Enhancement
Cholic Acid 3-O-β-Glucuronide Disodium Salt, as a type of bile acid (BA), has been used to enhance milk quality and modulate the gut microbiota of dairy goats . BAs supplementation has shown to positively influence milk yield and improve the quality of fatty acids in goat milk . It led to a reduction in saturated fatty acids (C16:0) and an increase in monounsaturated fatty acids (cis-9 C18:1), resulting in a healthier milk fatty acid profile .
Lipid Metabolism
BAs play a crucial role in lipid metabolism, acting as emulsifiers and enhancers of lipid digestion and absorption in the small intestine . They can potentially modulate lipid-related processes .
Gut Microbiota Modulation
BAs supplementation has been found to induce significant changes in the composition of the gut microbiota, favoring the enrichment of specific bacterial groups and altering the balance of microbial populations . Correlation analysis revealed associations between specific bacterial groups (Bacillus and Christensenellaceae R-7 group) and BA types, suggesting a role for the gut microbiota in BA metabolism .
Drug Impurity Reference
Cholic Acid 3-O-β-Glucuronide Disodium Salt can be used as a reference substance for drug impurities . This application is particularly useful in pharmaceutical research and development.
Nutrient Digestion
BAs, including Cholic Acid 3-O-β-Glucuronide Disodium Salt, are known to aid in the digestion of nutrients . They act as emulsifiers, helping to break down fats and enhance their absorption in the small intestine .
Therapeutic Applications
BAs have potential therapeutic applications in various diseases such as primary biliary cirrhosis (PBC), primary sclerosing cholangitis, gallstones, digestive tract diseases, cystic fibrosis, and cancer . Further research is needed to explore these applications in more detail.
properties
{ "Design of the Synthesis Pathway": "The synthesis pathway of Cholic Acid 3-O-β-Glucuronide Disodium Salt involves the glucuronidation of cholic acid using glucuronic acid and subsequent disodium salt formation.", "Starting Materials": [ "Cholic acid", "Glucuronic acid", "Sodium hydroxide", "Sodium chloride", "Water" ], "Reaction": [ "Cholic acid is dissolved in water and sodium hydroxide is added to adjust the pH to 8-9.", "Glucuronic acid is added to the solution and the mixture is stirred at room temperature for 24 hours.", "The reaction mixture is then acidified with hydrochloric acid to pH 2-3.", "The resulting precipitate is filtered and washed with water.", "The crude product is dissolved in water and disodium hydrogen phosphate is added to adjust the pH to 7-8.", "The solution is then evaporated to dryness to obtain the Cholic Acid 3-O-β-Glucuronide Disodium Salt." ] } | |
CAS RN |
59274-69-0 |
Molecular Formula |
C₃₀H₄₆Na₂O₁₁ |
Molecular Weight |
628.66 |
synonyms |
β-D-Glucopyranosiduronic Acid Derivative; 24-Norcholane; (3α,5β,7α,12α)-23-Carboxy-7,12-dihydroxy-24-norcholan-3-yl, β-D-Glucopyranosiduronic Acid Disodium Salt |
Origin of Product |
United States |
Disclaimer and Information on In-Vitro Research Products
Please be aware that all articles and product information presented on BenchChem are intended solely for informational purposes. The products available for purchase on BenchChem are specifically designed for in-vitro studies, which are conducted outside of living organisms. In-vitro studies, derived from the Latin term "in glass," involve experiments performed in controlled laboratory settings using cells or tissues. It is important to note that these products are not categorized as medicines or drugs, and they have not received approval from the FDA for the prevention, treatment, or cure of any medical condition, ailment, or disease. We must emphasize that any form of bodily introduction of these products into humans or animals is strictly prohibited by law. It is essential to adhere to these guidelines to ensure compliance with legal and ethical standards in research and experimentation.