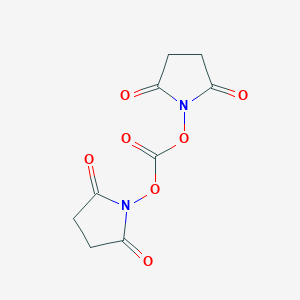
N,N'-Disuccinimidyl carbonate
Overview
Description
Mechanism of Action
Target of Action
Bis(2,5-dioxopyrrolidin-1-yl) carbonate, also known as N,N’-disuccinimidyl carbonate , is a chemical compound that primarily targets biomacromolecules . It falls within the category of reversible linkers used for biomacromolecule conjugation with active small molecules.
Mode of Action
The compound is a homobifunctional, amine-targeting, sulfoxide-containing crosslinker . It is used for the analysis of protein-protein interactions (PPIs) through crosslinking mass spectrometry (XL-MS) . It possesses two N-hydroxysuccinimide (NHS) esters for targeting Lys, a 10.1 Å spacer arm, and two symmetrical C-S cleavable bonds adjacent to the central sulfoxide .
Pharmacokinetics
It is known that the compound should be stored in an inert atmosphere at 2-8°c , suggesting that its stability and bioavailability may be temperature-dependent.
Result of Action
The result of the action of Bis(2,5-dioxopyrrolidin-1-yl) carbonate is the formation of crosslinks between proteins, enabling the study of protein-protein interactions . The post-cleavage spacer yields tagged peptides for unambiguous identification by collision-induced dissociation in tandem MS .
Action Environment
The compound should be used in a well-ventilated area to prevent concentration in hollows and sumps . It is also recommended to avoid all personal contact, including inhalation, and to wear protective clothing when risk of exposure occurs . These precautions suggest that the compound’s action, efficacy, and stability may be influenced by environmental factors such as ventilation and exposure conditions.
Biochemical Analysis
Biochemical Properties
Bis(2,5-dioxopyrrolidin-1-yl) carbonate plays a crucial role in biochemical reactions, particularly in the formation of covalent bonds between biomolecules. It interacts with enzymes, proteins, and other biomolecules through its reactive N-hydroxysuccinimide (NHS) ester groups. These groups target primary amines on lysine residues of proteins, facilitating the formation of stable amide bonds. This interaction is essential for crosslinking proteins, which helps in studying protein-protein interactions and protein complex structures .
Cellular Effects
Bis(2,5-dioxopyrrolidin-1-yl) carbonate influences various cellular processes by modifying proteins and altering their functions. It can affect cell signaling pathways, gene expression, and cellular metabolism by crosslinking proteins involved in these processes. For example, the crosslinking of signaling proteins can lead to changes in signal transduction pathways, impacting cellular responses to external stimuli. Additionally, the modification of transcription factors by Bis(2,5-dioxopyrrolidin-1-yl) carbonate can influence gene expression patterns .
Molecular Mechanism
The molecular mechanism of Bis(2,5-dioxopyrrolidin-1-yl) carbonate involves the formation of covalent bonds with primary amines on proteins. This reaction is facilitated by the NHS ester groups, which react with the amine groups to form stable amide bonds. This crosslinking process can inhibit or activate enzymes, depending on the specific proteins involved. For instance, the crosslinking of enzymes can prevent substrate binding, leading to enzyme inhibition. Conversely, crosslinking can also stabilize enzyme-substrate complexes, enhancing enzymatic activity .
Temporal Effects in Laboratory Settings
In laboratory settings, the effects of Bis(2,5-dioxopyrrolidin-1-yl) carbonate can change over time due to its stability and degradation properties. The compound is generally stable under controlled conditions, but it can degrade over time, especially in the presence of moisture. Long-term studies have shown that the crosslinking effects of Bis(2,5-dioxopyrrolidin-1-yl) carbonate can persist, leading to sustained modifications of proteins and prolonged changes in cellular functions .
Dosage Effects in Animal Models
The effects of Bis(2,5-dioxopyrrolidin-1-yl) carbonate in animal models vary with different dosages. At low doses, the compound can effectively crosslink proteins without causing significant toxicity. At high doses, it can lead to adverse effects, including cytotoxicity and tissue damage. Studies have shown that there is a threshold dose beyond which the toxic effects become pronounced, highlighting the importance of dosage optimization in experimental settings .
Metabolic Pathways
Bis(2,5-dioxopyrrolidin-1-yl) carbonate is involved in metabolic pathways that include the modification of proteins and peptides. It interacts with enzymes and cofactors that facilitate the crosslinking process. The compound can affect metabolic flux by altering the activity of enzymes involved in metabolic pathways. For example, the crosslinking of metabolic enzymes can lead to changes in metabolite levels and shifts in metabolic flux .
Transport and Distribution
Within cells and tissues, Bis(2,5-dioxopyrrolidin-1-yl) carbonate is transported and distributed through interactions with transporters and binding proteins. These interactions influence the localization and accumulation of the compound in specific cellular compartments. The distribution of Bis(2,5-dioxopyrrolidin-1-yl) carbonate can affect its activity and function, as it needs to reach target proteins to exert its crosslinking effects .
Subcellular Localization
The subcellular localization of Bis(2,5-dioxopyrrolidin-1-yl) carbonate is determined by targeting signals and post-translational modifications. The compound can be directed to specific compartments or organelles, where it can modify proteins and influence their functions. For example, Bis(2,5-dioxopyrrolidin-1-yl) carbonate can localize to the nucleus to crosslink transcription factors, affecting gene expression. Similarly, it can target mitochondria to modify metabolic enzymes .
Preparation Methods
Synthetic Routes and Reaction Conditions
N,N'-Disuccinimidyl carbonate can be synthesized through the reaction of phosgene with N-hydroxysuccinimide in the presence of a base such as triethylamine . The reaction is typically carried out in an inert solvent like dichloromethane at low temperatures to control the exothermic nature of the reaction .
Industrial Production Methods
In industrial settings, the production of bis(2,5-dioxopyrrolidin-1-yl) carbonate involves large-scale batch reactors where phosgene and N-hydroxysuccinimide are reacted under controlled conditions. The product is then purified through recrystallization or other suitable purification techniques to achieve the desired purity .
Chemical Reactions Analysis
Types of Reactions
N,N'-Disuccinimidyl carbonate primarily undergoes substitution reactions where the carbonate group is replaced by nucleophiles such as amines or alcohols . It does not typically undergo oxidation or reduction reactions due to its stable carbonate structure .
Common Reagents and Conditions
Common reagents used in reactions with bis(2,5-dioxopyrrolidin-1-yl) carbonate include primary and secondary amines, alcohols, and thiols . These reactions are usually carried out in polar aprotic solvents like dimethylformamide (DMF) or dichloromethane at room temperature .
Major Products Formed
The major products formed from reactions with bis(2,5-dioxopyrrolidin-1-yl) carbonate are N-hydroxysuccinimide esters, which are valuable intermediates in organic synthesis and pharmaceutical development .
Scientific Research Applications
N,N'-Disuccinimidyl carbonate has a wide range of applications in scientific research:
Comparison with Similar Compounds
Similar Compounds
Bis(2,5-dioxopyrrolidin-1-yl) 3,3’-sulfinyldipropionate: Similar in structure but contains a sulfoxide group, making it useful for studying protein-protein interactions.
Benzyl 2,5-dioxopyrrolidin-1-yl carbonate: Used as a mild reagent for protecting amino acids.
Uniqueness
N,N'-Disuccinimidyl carbonate is unique due to its high reactivity and specificity for hydroxyl group activation. Its stability and ease of handling make it a preferred reagent in both laboratory and industrial settings .
Biological Activity
N,N'-Disuccinimidyl carbonate (DSC) is a versatile reagent widely used in bioconjugation chemistry, particularly for the modification of proteins and other biomolecules. This article explores its biological activity, applications, and relevant research findings, supported by data tables and case studies.
Overview of this compound
This compound is an activated carbonate compound that facilitates the coupling of various ligands to amines, particularly in protein engineering and drug development. Its ability to form stable carbamate bonds makes it a valuable tool in synthesizing biologically active molecules.
DSC acts as a coupling agent through the formation of N-hydroxysuccinimide (NHS) esters, which can react with primary amines to yield stable carbamates. This reaction is advantageous for modifying proteins without significantly altering their biological activity.
Applications in Bioconjugation
- Protein Modification : DSC is utilized for the selective modification of lysine residues on proteins. This method allows for the attachment of various functional groups or labels, enhancing the protein's utility in research and therapeutic applications .
- Synthesis of Prodrugs : DSC has been employed in synthesizing prodrugs that can be activated by specific enzymes, such as beta-glucuronidase. For instance, the reaction of beta-glucuronic acid glycosides with DSC has been shown to produce carbamates that can restore peptide binding to MHC molecules upon enzymatic activation .
- Affinity Chromatography : The compound is also used for functionalizing polysaccharides in affinity chromatography, improving the specificity and efficiency of protein purification processes .
Case Study: Alkoxycarbonylation of Amines
A study demonstrated the effectiveness of DSC in alkoxycarbonylation reactions, where various alcohols were reacted with amines to form carbamates. The yields from these reactions were generally high, indicating the reagent's efficiency:
Entry | Alcohol | Amine | Carbamate | Yield (%) |
---|---|---|---|---|
1 | 4 | 86 | 83 | |
2 | 2 | 6 | 65 | |
3 | 4 | 77 | 78 | |
4 | 8 | 6 | 72 | |
5 | 4 | 89 | 75 |
This table summarizes the yields achieved using DSC for various combinations of alcohols and amines, highlighting its versatility as a reagent .
Solubility Studies
Research into the solubility of DSC revealed that it has low solubility in many common organic solvents but is soluble in dimethyl sulfoxide (DMSO). This property affects its application in functionalization reactions, particularly when excess DSC needs to be removed after coupling reactions .
Properties
IUPAC Name |
bis(2,5-dioxopyrrolidin-1-yl) carbonate | |
---|---|---|
Source | PubChem | |
URL | https://pubchem.ncbi.nlm.nih.gov | |
Description | Data deposited in or computed by PubChem | |
InChI |
InChI=1S/C9H8N2O7/c12-5-1-2-6(13)10(5)17-9(16)18-11-7(14)3-4-8(11)15/h1-4H2 | |
Source | PubChem | |
URL | https://pubchem.ncbi.nlm.nih.gov | |
Description | Data deposited in or computed by PubChem | |
InChI Key |
PFYXSUNOLOJMDX-UHFFFAOYSA-N | |
Source | PubChem | |
URL | https://pubchem.ncbi.nlm.nih.gov | |
Description | Data deposited in or computed by PubChem | |
Canonical SMILES |
C1CC(=O)N(C1=O)OC(=O)ON2C(=O)CCC2=O | |
Source | PubChem | |
URL | https://pubchem.ncbi.nlm.nih.gov | |
Description | Data deposited in or computed by PubChem | |
Molecular Formula |
C9H8N2O7 | |
Source | PubChem | |
URL | https://pubchem.ncbi.nlm.nih.gov | |
Description | Data deposited in or computed by PubChem | |
DSSTOX Substance ID |
DTXSID10225080 | |
Record name | Di(succinimido) carbonate | |
Source | EPA DSSTox | |
URL | https://comptox.epa.gov/dashboard/DTXSID10225080 | |
Description | DSSTox provides a high quality public chemistry resource for supporting improved predictive toxicology. | |
Molecular Weight |
256.17 g/mol | |
Source | PubChem | |
URL | https://pubchem.ncbi.nlm.nih.gov | |
Description | Data deposited in or computed by PubChem | |
CAS No. |
74124-79-1 | |
Record name | Disuccinimidyl carbonate | |
Source | CAS Common Chemistry | |
URL | https://commonchemistry.cas.org/detail?cas_rn=74124-79-1 | |
Description | CAS Common Chemistry is an open community resource for accessing chemical information. Nearly 500,000 chemical substances from CAS REGISTRY cover areas of community interest, including common and frequently regulated chemicals, and those relevant to high school and undergraduate chemistry classes. This chemical information, curated by our expert scientists, is provided in alignment with our mission as a division of the American Chemical Society. | |
Explanation | The data from CAS Common Chemistry is provided under a CC-BY-NC 4.0 license, unless otherwise stated. | |
Record name | Di(succinimido) carbonate | |
Source | ChemIDplus | |
URL | https://pubchem.ncbi.nlm.nih.gov/substance/?source=chemidplus&sourceid=0074124791 | |
Description | ChemIDplus is a free, web search system that provides access to the structure and nomenclature authority files used for the identification of chemical substances cited in National Library of Medicine (NLM) databases, including the TOXNET system. | |
Record name | Di(succinimido) carbonate | |
Source | EPA DSSTox | |
URL | https://comptox.epa.gov/dashboard/DTXSID10225080 | |
Description | DSSTox provides a high quality public chemistry resource for supporting improved predictive toxicology. | |
Record name | Di(succinimido) carbonate | |
Source | European Chemicals Agency (ECHA) | |
URL | https://echa.europa.eu/substance-information/-/substanceinfo/100.070.643 | |
Description | The European Chemicals Agency (ECHA) is an agency of the European Union which is the driving force among regulatory authorities in implementing the EU's groundbreaking chemicals legislation for the benefit of human health and the environment as well as for innovation and competitiveness. | |
Explanation | Use of the information, documents and data from the ECHA website is subject to the terms and conditions of this Legal Notice, and subject to other binding limitations provided for under applicable law, the information, documents and data made available on the ECHA website may be reproduced, distributed and/or used, totally or in part, for non-commercial purposes provided that ECHA is acknowledged as the source: "Source: European Chemicals Agency, http://echa.europa.eu/". Such acknowledgement must be included in each copy of the material. ECHA permits and encourages organisations and individuals to create links to the ECHA website under the following cumulative conditions: Links can only be made to webpages that provide a link to the Legal Notice page. | |
Synthesis routes and methods
Procedure details
Retrosynthesis Analysis
AI-Powered Synthesis Planning: Our tool employs the Template_relevance Pistachio, Template_relevance Bkms_metabolic, Template_relevance Pistachio_ringbreaker, Template_relevance Reaxys, Template_relevance Reaxys_biocatalysis model, leveraging a vast database of chemical reactions to predict feasible synthetic routes.
One-Step Synthesis Focus: Specifically designed for one-step synthesis, it provides concise and direct routes for your target compounds, streamlining the synthesis process.
Accurate Predictions: Utilizing the extensive PISTACHIO, BKMS_METABOLIC, PISTACHIO_RINGBREAKER, REAXYS, REAXYS_BIOCATALYSIS database, our tool offers high-accuracy predictions, reflecting the latest in chemical research and data.
Strategy Settings
Precursor scoring | Relevance Heuristic |
---|---|
Min. plausibility | 0.01 |
Model | Template_relevance |
Template Set | Pistachio/Bkms_metabolic/Pistachio_ringbreaker/Reaxys/Reaxys_biocatalysis |
Top-N result to add to graph | 6 |
Feasible Synthetic Routes
Disclaimer and Information on In-Vitro Research Products
Please be aware that all articles and product information presented on BenchChem are intended solely for informational purposes. The products available for purchase on BenchChem are specifically designed for in-vitro studies, which are conducted outside of living organisms. In-vitro studies, derived from the Latin term "in glass," involve experiments performed in controlled laboratory settings using cells or tissues. It is important to note that these products are not categorized as medicines or drugs, and they have not received approval from the FDA for the prevention, treatment, or cure of any medical condition, ailment, or disease. We must emphasize that any form of bodily introduction of these products into humans or animals is strictly prohibited by law. It is essential to adhere to these guidelines to ensure compliance with legal and ethical standards in research and experimentation.