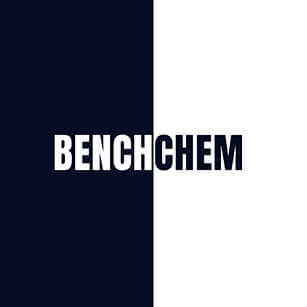
(Acetylacetonato)dicarbonylrhodium(I)
- Click on QUICK INQUIRY to receive a quote from our team of experts.
- With the quality product at a COMPETITIVE price, you can focus more on your research.
Overview
Description
(Acetylacetonato)dicarbonylrhodium(I), [Rh(acac)(CO)₂], is a Rh(I) complex with a square-planar geometry. Its molecular formula is Rh(CO)₂(C₅H₇O₂) (MW: 258.03 g/mol), and it exists as a red or deep green solid with a melting point of 154–156°C . The acetylacetonate (acac) ligand acts as a bidentate chelator, while the two CO ligands are labile, enabling dynamic ligand substitution during catalysis.
Preparation Methods
Traditional Synthesis Approaches
Early methods for synthesizing (acetylacetonato)dicarbonylrhodium(I) relied on direct reactions between rhodium salts and acetylacetone under carbon monoxide atmospheres. For example, CN102030781A describes refluxing rhodium chloride in alcoholic solutions with carbon monoxide, followed by acetylacetone addition . While achieving yields up to 93.9%, this approach introduced high chloride ion content (>1,500 ppm) due to residual RhCl₃, necessitating additional purification steps . The reaction mechanism involves ligand substitution, where CO and acetylacetonate displace chloride ions:
3 + 2\text{CO} + \text{acac}^- \rightarrow \text{Rh(CO)}2(\text{acac}) + 3\text{Cl}^-RhCl3+2CO+acac−→Rh(CO)2(acac)+3Cl−
However, incomplete chloride displacement and solvent choice (e.g., methanol) limited reproducibility, as highlighted by variable melting points and NMR spectra in early patents .
Advanced Precipitation-Based Methods
To address chloride contamination, CN103709201A and CN103709201B introduced a precipitation step using rhodium trichloride and agents like sodium hydroxide . This method involves:
-
Precipitation : Treating RhCl₃ with NaOH to form Rh(OH)₃, reducing free chloride ions.
-
Dissolution : Reacting the precipitate with dimethylformamide (DMF) at 140–160°C.
-
Ligand Addition : Introducing acetylacetone at a 9:1 molar ratio (acac:Rh) under nitrogen .
Table 1 compares outcomes from precipitation versus non-precipitation methods:
Parameter | Precipitation Method | Traditional Method |
---|---|---|
Yield (%) | 95–98 | 85–90 |
Cl⁻ Content (ppm) | <200 | >1,500 |
Reaction Time (min) | 50–90 | 60–120 |
This approach reduces chloride content by 87% and enhances yield through optimized ligand ratios and solvent selection .
Solvent and Reaction Condition Optimization
CN102372744A emphasizes solvent composition, particularly water content in DMF, to stabilize intermediates . Key findings include:
-
Water Content : Maintaining 0.5% water in DMF prevents rhodium aggregation, ensuring uniform reaction kinetics.
-
Temperature : Reactions at 148±2°C achieve complete RhCl₃ dissolution within 30 minutes, versus >60 minutes at lower temperatures .
Table 2 illustrates water-to-reaction mixture ratios and their impact:
Excess water (>8:1) precipitates unreacted RhCl₃, lowering yields, while insufficient water (<4:1) causes side reactions .
Post-Synthesis Processing and Purification
Post-synthesis steps critically influence product purity:
-
Crystallization : Recrystallization in n-hexane removes residual DMF and acetylacetone, yielding >99% purity .
-
Drying : Vacuum drying at 25°C prevents thermal decomposition, preserving carbonyl ligands .
CN114573640A introduces aromatic aldehydes (e.g., benzaldehyde) to sequester unreacted acetylacetone, simplifying filtration . Post-treatment with ethanol reduces particle size, enhancing catalytic surface area in downstream applications .
Comparative Analysis of Methodologies
Table 3 synthesizes data across methodologies:
Method | Key Advantage | Limitation |
---|---|---|
Precipitation | Low Cl⁻, high yield | Multi-step, costly |
Solvent-Optimized | Rapid kinetics | Sensitive to H₂O content |
Aldehyde-Assisted | Simplified purification | Lower yield (80–85%) |
Industrial scalability favors precipitation methods despite higher operational complexity, as chloride limits catalyst poisoning in hydroformylation .
Industrial Applications and Scalability
(Acetylacetonato)dicarbonylrhodium(I) derivatives, such as acetylacetonatotriphenylphosphinerhodium, are pivotal in propylene hydroformylation. CN103709201B demonstrates scaling to 50 kg batches using continuous CO flow and automated pH control, achieving 96% yield with 180 ppm Cl⁻ . However, DMF recycling remains challenging due to azeotrope formation with water, necessitating molecular sieve adsorption .
Chemical Reactions Analysis
Types of Reactions
(Acetylacetonato)dicarbonylrhodium(I) undergoes various types of chemical reactions, including:
Oxidation: The compound can be oxidized to form higher oxidation state rhodium complexes.
Reduction: It can be reduced to form lower oxidation state rhodium species.
Substitution: The carbonyl and acetylacetonate ligands can be substituted by other ligands, such as phosphines or amines.
Addition: The compound can participate in addition reactions with unsaturated substrates, such as alkenes and alkynes.
Common Reagents and Conditions
Common reagents used in reactions with (Acetylacetonato)dicarbonylrhodium(I) include:
Oxidizing agents: Hydrogen peroxide, oxygen
Reducing agents: Sodium borohydride, lithium aluminum hydride
Ligands: Triphenylphosphine, pyridine
Solvents: Toluene, dichloromethane, tetrahydrofuran
Major Products
The major products formed from reactions with (Acetylacetonato)dicarbonylrhodium(I) depend on the specific reaction conditions and reagents used. For example, substitution reactions with phosphines can yield rhodium-phosphine complexes, while addition reactions with alkenes can produce rhodium-alkene adducts .
Scientific Research Applications
(Acetylacetonato)dicarbonylrhodium(I) has a wide range of scientific research applications, including:
Catalysis: It is used as a catalyst or catalyst precursor in various catalytic processes, such as hydroformylation, carbonylation, and hydrogenation reactions. Its ability to activate and transfer functional groups makes it valuable in the synthesis of fine chemicals and pharmaceuticals.
Mechanistic Studies: The compound is employed in mechanistic studies to understand the fundamental steps involved in metal-mediated transformations. Researchers use it to investigate reaction kinetics and develop improved catalyst systems.
Material Science: It is used in the preparation of rhodium-containing materials with unique electronic and optical properties
Biological Studies: Although less common, (Acetylacetonato)dicarbonylrhodium(I) is explored for its potential biological activity and interactions with biomolecules .
Mechanism of Action
The mechanism of action of (Acetylacetonato)dicarbonylrhodium(I) involves the coordination of the rhodium center with various substrates, facilitating their activation and transformation. The carbonyl and acetylacetonate ligands play a crucial role in stabilizing the rhodium center and modulating its reactivity. The compound can undergo ligand exchange, oxidative addition, and reductive elimination processes, which are key steps in many catalytic cycles. The molecular targets and pathways involved depend on the specific reaction and substrate being studied .
Comparison with Similar Compounds
Comparison with Similar Rhodium(I) Complexes
Ligand Variations and Structural Differences
† Benzoyl-1,1,1-trifluoroacetonatodicarbonylrhodium(I)
‡ Acetylacetonato(1,5-cyclooctadiene)rhodium(I)
Structural Implications :
- CO vs. Phosphine Ligands: Phosphine ligands (e.g., PPh₃) are stronger σ-donors than CO, increasing electron density at the Rh center, which can alter catalytic selectivity .
- Acac Derivatives : Electron-withdrawing substituents (e.g., trifluoro groups in bz-tfac) enhance electrophilicity, favoring oxidative addition steps .
Catalytic Performance
Hydroformylation Activity
Key Findings :
- [Rh(acac)(CO)₂] achieves higher TOF under industrial pressures (100 bar) due to CO ligand lability, facilitating active species formation .
- Phosphine-containing complexes (e.g., [Rh(acac)(CO)(PPh₃)]) exhibit lower activity but improved regioselectivity in hydroformylation .
Stability and Ligand Substitution Dynamics
- CO Ligand Lability : In [Rh(acac)(CO)₂], CO ligands are replaced by Et₃N under reaction conditions, as shown by in situ XANES/EXAFS . This dynamic behavior is critical for catalytic cycles.
- Phosphine Stability : Complexes like [Rh(acac)(CO)(PPh₃)] resist ligand substitution, making them less adaptable but more stable in low-pressure systems .
Electrochemical Properties
Compound | Reduction Potential (V vs. SCE) | Oxidation Potential (V vs. SCE) | Reference |
---|---|---|---|
[Rh(acac)(CO)₂] | -1.2 | +0.8 | |
[Rh(acac)(COD)] | -0.9 | +1.1 |
Implications: Lower reduction potentials in COD-containing complexes suggest easier oxidation, aligning with their use in hydrogenation reactions .
Biological Activity
(Acetylacetonato)dicarbonylrhodium(I), commonly known as Rh(acac)(CO)₂, is an organorhodium compound that has garnered significant interest in both catalysis and biological applications. This compound is characterized by its unique structure, which consists of two carbon monoxide (CO) ligands and an acetylacetonate ligand, contributing to its catalytic properties in various chemical reactions. This article explores the biological activity of this compound, including its mechanisms of action, potential therapeutic applications, and relevant research findings.
- Molecular Formula : Rh(C₅H₇O₂)₂(CO)₂
- Molecular Weight : 258.03 g/mol
- Appearance : Dark green solid that dissolves in organic solvents like acetone and benzene, yielding yellow solutions.
Biological Activity Overview
Research into the biological activity of (Acetylacetonato)dicarbonylrhodium(I) focuses primarily on its role as a catalyst in organic synthesis and its potential implications in medicinal chemistry. The compound has been studied for its ability to facilitate various reactions that could lead to the development of pharmaceutical agents.
- Catalytic Activity : Rh(acac)(CO)₂ acts as a catalyst in hydroformylation and carbonylation reactions, which are crucial for synthesizing aldehydes and other functional groups relevant in drug development .
- Metal-Mediated Transformations : The compound is involved in metal-mediated transformations that can enhance the reactivity of substrates, making it valuable for synthesizing complex organic molecules .
Case Studies
- Hydroformylation of Olefins : A study demonstrated that (Acetylacetonato)dicarbonylrhodium(I) effectively catalyzes the hydroformylation of olefins, producing valuable aldehyde products. This reaction is significant for producing intermediates used in pharmaceuticals .
- Silylformylation Reactions : The compound has been utilized in silylformylation reactions, showcasing its versatility in forming carbon-silicon bonds, which are essential in organic synthesis .
- Conjugate Additions : Research indicates that Rh(acac)(CO)₂ facilitates conjugate additions of aryl- and alkenylboronic acids to enones, further expanding its utility in creating diverse chemical scaffolds for drug discovery .
Data Tables
Q & A
Basic Research Questions
Q. What are the common synthetic routes for (Acetylacetonato)dicarbonylrhodium(I), and how is purity assessed?
- Methodological Answer : The compound is typically synthesized via the reaction of rhodium(III) chloride (RhCl₃) with carbon monoxide (CO) and acetylacetone under controlled conditions. Purification involves recrystallization from organic solvents. Purity is assessed using melting point analysis (154–156°C, lit.) and assay data (98% purity via titration or chromatography) . Key parameters include reaction temperature (maintained at 50–80°C) and CO pressure.
Q. Which spectroscopic techniques are employed to characterize (Acetylacetonato)dicarbonylrhodium(I)?
- Methodological Answer :
- IR Spectroscopy : Identifies ν(CO) stretching frequencies (1900–2100 cm⁻¹), confirming the presence of dicarbonyl ligands.
- NMR Spectroscopy : Probes the acetylacetonato (acac) ligand’s electronic environment (e.g., ¹H NMR for methyl and methine protons).
- X-ray Crystallography : Resolves the octahedral geometry at Rh(I), with bond lengths (Rh–C: ~1.8 Å) and angles validated against the SMILES string ([C-]#[O+].[C-]#[O+].CC(=O)\C=C(\C)O[Rh]) .
Q. What safety protocols are critical when handling this compound?
- Methodological Answer :
- Hazards : Flammable solid (H228), skin sensitization (H317), and eye irritation (H319).
- PPE : N95 masks, gloves, and eyeshields.
- Storage : Class 4.1B (flammable solids), stored in airtight containers away from ignition sources .
Q. How does the electronic structure of Rh(I) influence its catalytic activity?
- Methodological Answer : The low oxidation state (Rh⁺) and strong-field CO ligands stabilize a 16-electron configuration, enhancing substrate binding in catalytic cycles. The acac ligand’s π-donor properties modulate electron density at Rh, affecting turnover rates in hydroformylation .
Advanced Research Questions
Q. What challenges arise in optimizing solvent systems for fluorous-phase hydroformylation using this catalyst?
- Methodological Answer : Fluorous solvents (e.g., perfluorohydrocarbons) improve catalyst recovery but require precise solubility studies. Variables include partition coefficients and CO diffusion rates. Conflicting reports on efficiency may stem from solvent polarity effects on Rh–CO bond stability .
Q. How can computational models (e.g., DFT) elucidate the reaction mechanism of this complex in C–H activation?
- Methodological Answer : Density Functional Theory (DFT) calculates electron density maps and transition states. Key inputs: Rh’s d⁸ configuration (molecular weight: 258.03 g/mol) and CO ligand bond orders. Discrepancies between theoretical and experimental turnover numbers often relate to solvent interactions omitted in simulations .
Q. What strategies resolve contradictions in reported catalytic activity for asymmetric hydroformylation?
- Methodological Answer :
- Variable Control : Compare substrate scope, pressure (CO/H₂), and temperature (lit. range: 60–120°C).
- Data Normalization : Express activity as turnover frequency (TOF) rather than yield.
- Spectroscopic Validation : In-situ IR monitors CO ligand loss during deactivation .
Q. How do steric modifications of the acac ligand impact catalytic selectivity?
- Methodological Answer : Substitute methyl groups in acac with bulkier substituents (e.g., tert-butyl). Compare crystallographic data (bond angles) and catalytic outcomes. Advanced NMR (NOESY) probes ligand flexibility, correlating with enantioselectivity in chiral products .
Q. Key Research Considerations
- Novelty : Explore understudied substrates (e.g., alkenes with electron-withdrawing groups).
- Ethics : Adhere to waste disposal protocols for Rh (heavy metal).
- Feasibility : Pilot studies using <100 mg of catalyst to minimize costs.
Properties
CAS No. |
14874-82-9 |
---|---|
Molecular Formula |
C₇H₇O₄Rh |
Molecular Weight |
258.03 |
Synonyms |
Acetylacetonatorhodium Dicarbonyl; Dicarbonyl(2,4-pentanedionato)rhodium; Dicarbonyl(2,4-pentanedionato-κO,κO’)rhodium; Dicarbonylacetylacetonatorhodium; Dicarbonylacetylacetonatorhodium(I); Dicarbonylrhodium Acetylacetonate; Dicarbonylrhodium(I) Ace |
Origin of Product |
United States |
Disclaimer and Information on In-Vitro Research Products
Please be aware that all articles and product information presented on BenchChem are intended solely for informational purposes. The products available for purchase on BenchChem are specifically designed for in-vitro studies, which are conducted outside of living organisms. In-vitro studies, derived from the Latin term "in glass," involve experiments performed in controlled laboratory settings using cells or tissues. It is important to note that these products are not categorized as medicines or drugs, and they have not received approval from the FDA for the prevention, treatment, or cure of any medical condition, ailment, or disease. We must emphasize that any form of bodily introduction of these products into humans or animals is strictly prohibited by law. It is essential to adhere to these guidelines to ensure compliance with legal and ethical standards in research and experimentation.