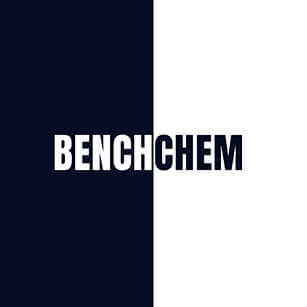
Amiodarone-d10 Hydrochloride
- Click on QUICK INQUIRY to receive a quote from our team of experts.
- With the quality product at a COMPETITIVE price, you can focus more on your research.
Overview
Description
Amiodarone-d10 Hydrochloride is a deuterated form of Amiodarone Hydrochloride, an antiarrhythmic agent used primarily for the treatment of various types of cardiac arrhythmias. The deuterium labeling in this compound enhances its stability and allows for more precise pharmacokinetic studies. This compound is particularly valuable in research settings where isotopic labeling is required to trace the metabolic pathways and interactions of the drug.
Mechanism of Action
Target of Action
Amiodarone-d10 Hydrochloride primarily targets the ATP-sensitive potassium channel . This channel plays a crucial role in the repolarization of the heart muscle during the third phase of the cardiac action potential .
Mode of Action
This compound is a class III anti-arrhythmic drug . It works by blocking the potassium currents that cause repolarization of the heart muscle during the third phase of the cardiac action potential . As a result, this compound increases the duration of the action potential as well as the effective refractory period for cardiac cells .
Biochemical Pathways
This compound affects multiple biochemical pathways. It inhibits both inward and outward currents . The inhibition of inward sodium and calcium currents (INa, ICa) is enhanced in a use- and voltage-dependent manner, resulting in suppression of excitability and conductivity of cardiac tissues . Both voltage- and ligand-gated potassium channel currents (IK, IK,Na, IK,ACh) are also inhibited at therapeutic levels of drug concentrations .
Pharmacokinetics
This compound exhibits complex pharmacokinetics. It accumulates in tissue and is only very slowly eliminated . The clearance of Amiodarone after intravenous administration in patients with ventricular fibrillation and ventricular tachycardia ranged from 220 to 440 ml/hr/kg . Another study determined that the total body clearance of Amiodarone varies from 0.10 to 0.77 L/min after one intravenous dose .
Result of Action
The major and consistent long-term effect of this compound is a moderate prolongation of the action potential duration (APD) with minimal frequency dependence . This prolongation is most likely due to a decrease in the current density of IK and Ito . Chronic Amiodarone was shown to cause a down-regulation of Kv1.5 messenger ribonucleic acid (mRNA) in rat hearts, suggesting a drug-induced modulation of potassium-channel gene expression .
Action Environment
The action of this compound can be influenced by environmental factors. Tissue accumulation of Amiodarone and its active metabolite (desethylamiodarone) may modulate the chronic effects, causing variable suppression of excitability and conductivity of the heart through the direct effects of the compounds retained at the sites of action . Amiodarone and desethylamiodarone could antagonize triiodothyronine (T3) action on the heart at cellular or subcellular levels, leading to phenotypic resemblance of long-term Amiodarone treatment and hypothyroidism .
Biochemical Analysis
Biochemical Properties
Amiodarone-d10 Hydrochloride plays a significant role in biochemical reactions, particularly in the modulation of ion channels in cardiac cells. It interacts with several enzymes, proteins, and other biomolecules. For instance, it inhibits the potassium channels responsible for repolarization of the heart muscle during the third phase of the cardiac action potential . Additionally, this compound interacts with sodium and calcium channels, as well as beta and alpha adrenergic receptors . These interactions result in the prolongation of the action potential duration and the effective refractory period for cardiac cells, thereby stabilizing cardiac rhythm.
Cellular Effects
This compound exerts various effects on different types of cells and cellular processes. In cardiac cells, it influences cell function by blocking potassium, sodium, and calcium channels, which affects the depolarization and repolarization phases of the cardiac action potential . This compound also impacts cell signaling pathways, gene expression, and cellular metabolism. For example, long-term use of this compound has been associated with changes in thyroid hormone metabolism, leading to alterations in gene expression related to thyroid function . Furthermore, it can cause cellular toxicity, particularly in the liver and lungs, due to its accumulation in these tissues .
Molecular Mechanism
The molecular mechanism of action of this compound involves multiple pathways. Primarily, it blocks potassium currents, which prolongs the repolarization phase of the cardiac action potential . It also inhibits inward sodium and calcium currents in a use- and voltage-dependent manner, resulting in the suppression of excitability and conductivity of cardiac tissues . Additionally, this compound can modulate gene expression by down-regulating potassium-channel gene expression, such as Kv1.5 messenger ribonucleic acid (mRNA) in rat hearts . This modulation leads to a decrease in the current density of potassium channels, further contributing to its antiarrhythmic effects.
Temporal Effects in Laboratory Settings
In laboratory settings, the effects of this compound change over time. The compound has a long half-life, ranging from 25 to 100 days, which results in prolonged effects even after discontinuation . Its stability and degradation are influenced by storage conditions, with studies showing that it remains stable for up to 90 days when stored in amber glass bottles at room temperature . Long-term use of this compound can lead to tissue accumulation, particularly in the liver, lungs, and thyroid, causing chronic toxicity .
Dosage Effects in Animal Models
The effects of this compound vary with different dosages in animal models. At therapeutic doses, it effectively manages arrhythmias without significant adverse effects . At higher doses, it can cause toxicity, including pulmonary fibrosis and liver damage . Studies have shown that the onset, peak, and duration of hypoglycemia are significantly increased when this compound is administered in combination with other drugs, indicating the need for careful dosage monitoring .
Metabolic Pathways
This compound is metabolized primarily in the liver, where it undergoes dealkylation to form its major metabolite, desethylamiodarone . This metabolite retains antiarrhythmic properties and contributes to the overall pharmacological effects of the compound. The metabolism of this compound can be influenced by other drugs, such as grapefruit juice, which inhibits its metabolism and leads to elevated drug levels . Additionally, chronic administration of this compound can modulate the expression of genes involved in potassium-channel regulation .
Transport and Distribution
This compound is highly protein-bound, with approximately 97% of the drug bound to plasma proteins . It has a large volume of distribution, indicating extensive tissue accumulation. The compound is transported within cells and tissues via passive diffusion and active transport mechanisms. It readily accumulates in tissues such as the liver, lungs, and thyroid, where it can exert its pharmacological and toxic effects . The distribution of this compound is influenced by its lipophilicity, allowing it to cross cell membranes and accumulate in lipid-rich tissues .
Subcellular Localization
The subcellular localization of this compound is primarily within the lysosomes and mitochondria of cells . This localization is facilitated by its lipophilic nature and the presence of targeting signals that direct it to specific compartments. Within the lysosomes, this compound can cause phospholipid accumulation, leading to cellular toxicity . In the mitochondria, it can disrupt mitochondrial function and energy production, contributing to its adverse effects on cellular metabolism .
Preparation Methods
Synthetic Routes and Reaction Conditions
The synthesis of Amiodarone-d10 Hydrochloride involves the incorporation of deuterium atoms into the molecular structure of Amiodarone Hydrochloride. This can be achieved through several synthetic routes, including:
Deuterium Exchange Reactions: This method involves the replacement of hydrogen atoms with deuterium in the presence of a deuterating agent such as deuterium oxide (D2O) or deuterated solvents.
Deuterated Reagents: Using deuterated reagents in the synthesis of Amiodarone can lead to the formation of Amiodarone-d10. For example, deuterated ethylamine can be used in the synthesis process to introduce deuterium atoms into the final product.
Industrial Production Methods
Industrial production of this compound typically involves large-scale deuterium exchange reactions under controlled conditions to ensure high yield and purity. The process may include:
Catalytic Deuteration: Utilizing catalysts such as palladium on carbon (Pd/C) to facilitate the exchange of hydrogen with deuterium.
Purification: The final product is purified using techniques such as recrystallization, chromatography, and distillation to achieve the desired isotopic purity.
Chemical Reactions Analysis
Types of Reactions
Amiodarone-d10 Hydrochloride undergoes various chemical reactions, including:
Oxidation: The compound can be oxidized in the presence of oxidizing agents such as hydrogen peroxide (H2O2) or potassium permanganate (KMnO4).
Reduction: Reduction reactions can be carried out using reducing agents like sodium borohydride (NaBH4) or lithium aluminum hydride (LiAlH4).
Substitution: Nucleophilic and electrophilic substitution reactions can occur, where functional groups in the molecule are replaced by other groups.
Common Reagents and Conditions
Oxidation: Hydrogen peroxide (H2O2), potassium permanganate (KMnO4), and chromium trioxide (CrO3) are commonly used oxidizing agents.
Reduction: Sodium borohydride (NaBH4) and lithium aluminum hydride (LiAlH4) are typical reducing agents.
Substitution: Halogenating agents such as thionyl chloride (SOCl2) and nucleophiles like sodium azide (NaN3) are used in substitution reactions.
Major Products Formed
The major products formed from these reactions depend on the specific conditions and reagents used. For example:
Oxidation: Oxidation of this compound can lead to the formation of hydroxylated or ketone derivatives.
Reduction: Reduction can result in the formation of deuterated alcohols or amines.
Substitution: Substitution reactions can produce various deuterated analogs with different functional groups.
Scientific Research Applications
Amiodarone-d10 Hydrochloride has a wide range of scientific research applications, including:
Pharmacokinetic Studies: The deuterium labeling allows for precise tracking of the drug’s absorption, distribution, metabolism, and excretion in the body.
Metabolic Pathway Analysis: Researchers use this compound to study the metabolic pathways and identify metabolites formed during drug metabolism.
Drug Interaction Studies: It is used to investigate potential interactions with other drugs and to understand the mechanisms of drug-drug interactions.
Isotope Dilution Mass Spectrometry: this compound serves as an internal standard in mass spectrometry for the quantification of Amiodarone and its metabolites.
Biomedical Research: The compound is used in studies related to cardiac arrhythmias, providing insights into the mechanisms of action and therapeutic effects of Amiodarone.
Comparison with Similar Compounds
Amiodarone-d10 Hydrochloride can be compared with other similar compounds, such as:
Dronedarone: A non-iodinated derivative of Amiodarone with similar antiarrhythmic properties but fewer side effects.
Sotalol: Another class III antiarrhythmic agent that also blocks potassium channels but lacks the deuterium labeling.
Ibutilide: A class III antiarrhythmic that prolongs the action potential duration but is used primarily for acute conversion of atrial fibrillation and flutter.
Uniqueness
The uniqueness of this compound lies in its deuterium labeling, which enhances its stability and allows for more precise pharmacokinetic and metabolic studies. This makes it a valuable tool in research settings where detailed analysis of drug behavior is required.
Properties
CAS No. |
1261393-77-4 |
---|---|
Molecular Formula |
C₂₅H₁₉D₁₀I₂NO₃·HCl |
Molecular Weight |
655.373646 |
Synonyms |
2-butyl-3-benzofuranyl 4-[2-(diethylamino)ethoxy]-3,5-diiodophenyl Ketone-d10; 2-Butyl-3-[3,5-diiodo-4-(2-diethylaminoethoxy)benzoyl]benzofuran-d10; 2-Butyl-3-benzofuranyl p-[(2-diethylamino)ethoxy]-m,m-diiodophenyl keto |
Origin of Product |
United States |
Disclaimer and Information on In-Vitro Research Products
Please be aware that all articles and product information presented on BenchChem are intended solely for informational purposes. The products available for purchase on BenchChem are specifically designed for in-vitro studies, which are conducted outside of living organisms. In-vitro studies, derived from the Latin term "in glass," involve experiments performed in controlled laboratory settings using cells or tissues. It is important to note that these products are not categorized as medicines or drugs, and they have not received approval from the FDA for the prevention, treatment, or cure of any medical condition, ailment, or disease. We must emphasize that any form of bodily introduction of these products into humans or animals is strictly prohibited by law. It is essential to adhere to these guidelines to ensure compliance with legal and ethical standards in research and experimentation.