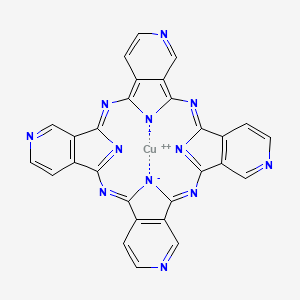
Copper(II) 4,4',4'',4'''-tetraaza-29H,31H-phthalocyanine
- Click on QUICK INQUIRY to receive a quote from our team of experts.
- With the quality product at a COMPETITIVE price, you can focus more on your research.
Overview
Description
Copper(II) 4,4’,4’‘,4’‘’-tetraaza-29H,31H-phthalocyanine is a coordination compound with the molecular formula C28H12CuN12. It is a member of the phthalocyanine family, which are macrocyclic compounds known for their intense color and stability. This compound is particularly notable for its applications in various fields due to its unique electronic properties and ability to form stable complexes with metals.
Mechanism of Action
Target of Action
Copper(II) 4,4’,4’‘,4’‘’-tetraaza-29H,31H-phthalocyanine primarily targets biological macromolecules such as DNA, proteins, and lipids. Its role involves interacting with these molecules to induce oxidative stress and disrupt cellular functions .
Mode of Action
The compound interacts with its targets through redox reactions . Copper(II) ions can undergo redox cycling, generating reactive oxygen species (ROS) such as superoxide anions and hydroxyl radicals. These ROS can cause oxidative damage to DNA, proteins, and lipids, leading to cellular dysfunction and apoptosis .
Biochemical Pathways
The generation of ROS by Copper(II) 4,4’,4’‘,4’‘’-tetraaza-29H,31H-phthalocyanine affects several biochemical pathways:
- Lipid Peroxidation: ROS can initiate lipid peroxidation, disrupting membrane integrity and signaling pathways .
Pharmacokinetics
The pharmacokinetics of Copper(II) 4,4’,4’‘,4’‘’-tetraaza-29H,31H-phthalocyanine involve its absorption, distribution, metabolism, and excretion (ADME) properties:
Result of Action
The molecular and cellular effects of Copper(II) 4,4’,4’‘,4’‘’-tetraaza-29H,31H-phthalocyanine include:
- Disruption of Cellular Homeostasis: The compound’s action leads to an imbalance in cellular redox states and metabolic processes .
Action Environment
Environmental factors significantly influence the compound’s action, efficacy, and stability:
- Oxygen Availability: The generation of ROS is dependent on the availability of molecular oxygen, influencing the compound’s oxidative effects .
: Information synthesized from various sources on the compound’s properties and mechanism of action.
Preparation Methods
Synthetic Routes and Reaction Conditions: Copper(II) 4,4’,4’‘,4’‘’-tetraaza-29H,31H-phthalocyanine can be synthesized through several methods. One common approach involves the cyclotetramerization of phthalonitrile in the presence of a copper salt, such as copper(II) chloride, under high-temperature conditions. The reaction typically requires a solvent like quinoline or dimethylformamide and is carried out at temperatures ranging from 180°C to 220°C.
Industrial Production Methods: In industrial settings, the synthesis of Copper(II) 4,4’,4’‘,4’‘’-tetraaza-29H,31H-phthalocyanine often involves large-scale cyclotetramerization processes. These processes are optimized for high yield and purity, utilizing continuous flow reactors and automated systems to maintain precise control over reaction conditions.
Types of Reactions:
Oxidation: Copper(II) 4,4’,4’‘,4’‘’-tetraaza-29H,31H-phthalocyanine can undergo oxidation reactions, often facilitated by strong oxidizing agents such as hydrogen peroxide or potassium permanganate.
Reduction: This compound can also be reduced, typically using reducing agents like sodium borohydride or hydrazine.
Common Reagents and Conditions:
Oxidation: Hydrogen peroxide, potassium permanganate; typically carried out in aqueous or organic solvents.
Reduction: Sodium borohydride, hydrazine; often performed in solvents like ethanol or methanol.
Substitution: Various halides or organometallic reagents; reactions are usually conducted under inert atmosphere conditions to prevent unwanted side reactions.
Major Products:
Oxidation: Oxidized derivatives of the phthalocyanine ring.
Reduction: Reduced forms of the compound, often with altered electronic properties.
Substitution: Functionalized phthalocyanine derivatives with diverse chemical functionalities.
Scientific Research Applications
Copper(II) 4,4’,4’‘,4’‘’-tetraaza-29H,31H-phthalocyanine has a wide range of applications in scientific research:
Chemistry: Used as a catalyst in various organic reactions, including oxidation and polymerization processes.
Biology: Investigated for its potential as a photosensitizer in photodynamic therapy for cancer treatment.
Medicine: Explored for its antimicrobial properties and potential use in drug delivery systems.
Industry: Utilized in the production of dyes, pigments, and electronic materials due to its stability and electronic properties.
Comparison with Similar Compounds
Copper phthalocyanine: Similar in structure but lacks the additional nitrogen atoms in the macrocyclic ring.
Iron phthalocyanine: Contains iron instead of copper, leading to different electronic and catalytic properties.
Zinc phthalocyanine: Zinc substitution results in altered photophysical properties, making it useful in different applications.
Uniqueness: Copper(II) 4,4’,4’‘,4’‘’-tetraaza-29H,31H-phthalocyanine is unique due to the presence of additional nitrogen atoms in the macrocyclic ring, which enhances its ability to form stable complexes and participate in diverse chemical reactions. This structural feature also contributes to its distinct electronic properties, making it suitable for specialized applications in catalysis, medicine, and materials science.
Properties
IUPAC Name |
copper;2,6,11,15,20,24,29,34,38,40-decaza-37,39-diazanidanonacyclo[28.6.1.13,10.112,19.121,28.04,9.013,18.022,27.031,36]tetraconta-1,3(40),4(9),5,7,10,12,14,16,18,20,22(27),23,25,28(38),29,31(36),32,34-nonadecaene |
Source
|
---|---|---|
Source | PubChem | |
URL | https://pubchem.ncbi.nlm.nih.gov | |
Description | Data deposited in or computed by PubChem | |
InChI |
InChI=1S/C28H12N12.Cu/c1-5-29-9-17-13(1)21-33-22-14-2-6-31-11-19(14)27(35-22)40-28-20-12-32-8-4-16(20)24(39-28)38-26-18-10-30-7-3-15(18)23(37-26)36-25(17)34-21;/h1-12H;/q-2;+2 |
Source
|
Source | PubChem | |
URL | https://pubchem.ncbi.nlm.nih.gov | |
Description | Data deposited in or computed by PubChem | |
InChI Key |
VHJDNKDQMUHOKI-UHFFFAOYSA-N |
Source
|
Source | PubChem | |
URL | https://pubchem.ncbi.nlm.nih.gov | |
Description | Data deposited in or computed by PubChem | |
Canonical SMILES |
C1=CN=CC2=C1C3=NC4=NC(=NC5=C6C=CN=CC6=C([N-]5)N=C7C8=C(C=NC=C8)C(=N7)N=C2[N-]3)C9=C4C=CN=C9.[Cu+2] |
Source
|
Source | PubChem | |
URL | https://pubchem.ncbi.nlm.nih.gov | |
Description | Data deposited in or computed by PubChem | |
Molecular Formula |
C28H12CuN12 |
Source
|
Source | PubChem | |
URL | https://pubchem.ncbi.nlm.nih.gov | |
Description | Data deposited in or computed by PubChem | |
Molecular Weight |
580.0 g/mol |
Source
|
Source | PubChem | |
URL | https://pubchem.ncbi.nlm.nih.gov | |
Description | Data deposited in or computed by PubChem | |
Disclaimer and Information on In-Vitro Research Products
Please be aware that all articles and product information presented on BenchChem are intended solely for informational purposes. The products available for purchase on BenchChem are specifically designed for in-vitro studies, which are conducted outside of living organisms. In-vitro studies, derived from the Latin term "in glass," involve experiments performed in controlled laboratory settings using cells or tissues. It is important to note that these products are not categorized as medicines or drugs, and they have not received approval from the FDA for the prevention, treatment, or cure of any medical condition, ailment, or disease. We must emphasize that any form of bodily introduction of these products into humans or animals is strictly prohibited by law. It is essential to adhere to these guidelines to ensure compliance with legal and ethical standards in research and experimentation.