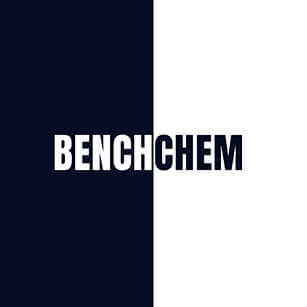
(±)-β-Santalene
- Click on QUICK INQUIRY to receive a quote from our team of experts.
- With the quality product at a COMPETITIVE price, you can focus more on your research.
Overview
Description
(±)-β-Santalene is a sesquiterpene hydrocarbon found in the essential oils of various plants, particularly in sandalwood. It is known for its woody and spicy aroma, making it a valuable component in the fragrance industry. The compound exists as a racemic mixture, meaning it contains equal amounts of both enantiomers.
Preparation Methods
Synthetic Routes and Reaction Conditions: The synthesis of (±)-β-Santalene typically involves the cyclization of farnesyl pyrophosphate, a common precursor in the biosynthesis of sesquiterpenes. This process can be catalyzed by specific enzymes known as sesquiterpene synthases. In a laboratory setting, the synthesis can be achieved through various organic reactions, including cyclization and rearrangement reactions under controlled conditions.
Industrial Production Methods: Industrial production of this compound often involves the extraction of essential oils from sandalwood trees, followed by purification processes such as distillation and chromatography. The yield and purity of this compound can be optimized through selective breeding of sandalwood trees and advanced extraction techniques.
Chemical Reactions Analysis
Types of Reactions: (±)-β-Santalene undergoes several types of chemical reactions, including:
Oxidation: This reaction can convert this compound into various oxygenated derivatives, such as alcohols, ketones, and aldehydes.
Reduction: Reduction reactions can be used to modify the double bonds in this compound, leading to the formation of saturated hydrocarbons.
Substitution: Substitution reactions can introduce different functional groups into the this compound molecule, altering its chemical properties.
Common Reagents and Conditions:
Oxidation: Common oxidizing agents include potassium permanganate, chromium trioxide, and hydrogen peroxide.
Reduction: Reducing agents such as lithium aluminum hydride and sodium borohydride are often used.
Substitution: Halogenation reactions can be carried out using reagents like chlorine or bromine under controlled conditions.
Major Products Formed: The major products formed from these reactions depend on the specific reagents and conditions used. For example, oxidation of this compound can yield santalol, a key component in sandalwood oil.
Scientific Research Applications
Table 1: Synthesis Methods for (±)-β-Santalene
Biological Properties
This compound exhibits several biological activities that make it a candidate for therapeutic applications:
- Anti-inflammatory Effects : Studies have demonstrated that sandalwood oil, rich in this compound, possesses anti-inflammatory properties, making it beneficial for treating skin conditions such as acne and eczema .
- Antimicrobial Activity : The compound has shown effectiveness against various pathogens, suggesting its potential use in developing antimicrobial agents .
- Anticancer Potential : Research indicates that this compound may possess anticancer properties, with studies exploring its effects on tumor cell lines .
Applications in Dermatology
The use of sandalwood oil containing this compound in dermatological formulations has gained traction due to its favorable safety profile and efficacy. Clinical trials have highlighted its benefits in treating inflammatory skin diseases. The compound's ability to modulate immune responses and reduce hyperplasia makes it a valuable ingredient in topical therapies .
Case Studies
- Sandalwood Oil for Acne Treatment : A clinical study investigated the efficacy of sandalwood oil containing this compound in patients with acne vulgaris. Results indicated significant reductions in lesion counts and inflammation after regular application over eight weeks .
- Efficacy Against Eczema : Another study assessed the use of sandalwood oil in patients with atopic dermatitis. The findings suggested that topical application led to improved skin hydration and reduced itching, attributed to the anti-inflammatory properties of this compound .
Table 2: Potential Future Applications of this compound
Application Area | Potential Use |
---|---|
Pharmaceuticals | Development of anti-inflammatory and antimicrobial drugs. |
Cosmetics | Formulations for skincare products targeting acne and eczema treatment. |
Aromatherapy | Use as a natural fragrance component with therapeutic benefits in stress relief and relaxation. |
Mechanism of Action
The mechanism of action of (±)-β-Santalene involves its interaction with various molecular targets and pathways. For instance, its antimicrobial activity is believed to result from its ability to disrupt microbial cell membranes. Additionally, its anti-inflammatory effects may be mediated through the inhibition of pro-inflammatory cytokines and enzymes.
Comparison with Similar Compounds
α-Santalene: Another sesquiterpene found in sandalwood oil, known for its similar woody aroma.
β-Santalol: An oxygenated derivative of (±)-β-Santalene, which is a major component of sandalwood oil and has similar applications in the fragrance industry.
Uniqueness: this compound is unique due to its specific chemical structure and the presence of both enantiomers in equal amounts. This racemic mixture contributes to its distinct aroma and chemical properties, differentiating it from other similar compounds.
Properties
CAS No. |
37876-50-9 |
---|---|
Molecular Formula |
C₁₅H₂₄ |
Molecular Weight |
204.35 |
Synonyms |
exo-2-Methyl-3-methylene-2-(4-methyl-3-pentenyl)-bicyclo[2.2.1]heptane; exo-(±)-2-methyl-3-methylene-2-(4-methyl-3-pentenyl)-bicyclo[2.2.1]heptane_x000B_ |
Origin of Product |
United States |
Q1: What is the molecular formula and weight of (±)-β-Santalene?
A1: this compound has a molecular formula of C15H24 and a molecular weight of 204.36 g/mol.
Q2: How can this compound be characterized spectroscopically?
A2: this compound can be characterized using techniques like Gas Chromatography-Mass Spectrometry (GC-MS) [, , , , , , , , , ] and Nuclear Magnetic Resonance (NMR) spectroscopy [, ]. GC-MS helps identify and quantify the compound based on its retention time and fragmentation pattern, while NMR provides structural information by analyzing the magnetic properties of its atomic nuclei.
Q3: What are the common starting materials for synthesizing this compound?
A3: Several synthetic routes have been explored for this compound, utilizing starting materials like norbornene [, ], camphor [, , , ], and endo-dicyclopentadiene []. The choice of starting material often dictates the stereoselectivity and efficiency of the synthesis.
Q4: Are there enantioselective syntheses for both enantiomers of β-Santalene?
A4: Yes, researchers have developed enantiodivergent routes to synthesize both (+) and (-) enantiomers of β-Santalene using a single chiral template []. This achievement is significant for studying the specific biological activities of each enantiomer.
Q5: How does the choice of catalyst affect the stereochemistry of the synthesized β-Santalene?
A5: The stereochemistry of the synthesized β-Santalene can be controlled by choosing appropriate chiral catalysts and reagents. For instance, using (+)- or (−)-camphor as a starting material allows access to either enantiomer of β-Santalene [].
Q6: What is the significance of developing an asymmetric Diels-Alder reaction for synthesizing this compound?
A6: Asymmetric Diels-Alder reactions offer a powerful tool for constructing the [2.2.1]bicycloheptane ring system found in this compound with high stereoselectivity [, , ]. This approach has been instrumental in achieving efficient and controlled syntheses of this valuable fragrance compound.
Q7: What is the primary application of this compound?
A7: this compound, as a component of sandalwood oil, is primarily used in the fragrance industry [, , , ]. Its woody, sweet aroma makes it a sought-after ingredient in perfumes, cosmetics, and incense.
Q8: Are there any known biological activities of this compound?
A8: While research on this compound's specific biological activities is limited, some studies suggest potential antifungal properties associated with sandalwood oil, of which it is a constituent []. Further research is needed to explore its potential therapeutic benefits.
Q9: How is this compound biosynthesized in nature?
A9: this compound biosynthesis in sandalwood trees involves specialized enzymes called terpene synthases [, ]. These enzymes catalyze the cyclization of farnesyl pyrophosphate (FPP), a precursor molecule derived from the isoprenoid pathway, to form this compound and other related sesquiterpenes.
Q10: What is the role of santalene synthases in the biosynthesis of sandalwood oil?
A10: Santalene synthases are key enzymes responsible for the diversity of sesquiterpenes found in sandalwood oil []. Different sandalwood species express distinct santalene synthases, leading to variations in the oil's chemical profile and contributing to the unique aroma profiles of different sandalwood species.
Q11: Can metabolic engineering be used to produce this compound sustainably?
A11: Research has explored the use of metabolic engineering to produce sandalwood fragrance compounds, including santalols and bergamotol, in engineered yeast cells []. This approach holds promise for a more sustainable and controlled production of sandalwood oil components, potentially alleviating the pressure on natural sandalwood resources.
Q12: Does this compound play a role in the chemical ecology of sandalwood trees?
A12: While the specific ecological role of this compound is not fully understood, its presence in sandalwood oil suggests potential functions in attracting pollinators or deterring herbivores. Further research is needed to elucidate its ecological significance.
Q13: Has the environmental impact of extracting this compound from natural sources been assessed?
A13: Sandalwood is a slow-growing tree species, and overharvesting for its valuable oil has raised concerns about sustainability. Research into alternative production methods, such as metabolic engineering [], aims to address these concerns and mitigate the environmental impact of sandalwood oil extraction.
Disclaimer and Information on In-Vitro Research Products
Please be aware that all articles and product information presented on BenchChem are intended solely for informational purposes. The products available for purchase on BenchChem are specifically designed for in-vitro studies, which are conducted outside of living organisms. In-vitro studies, derived from the Latin term "in glass," involve experiments performed in controlled laboratory settings using cells or tissues. It is important to note that these products are not categorized as medicines or drugs, and they have not received approval from the FDA for the prevention, treatment, or cure of any medical condition, ailment, or disease. We must emphasize that any form of bodily introduction of these products into humans or animals is strictly prohibited by law. It is essential to adhere to these guidelines to ensure compliance with legal and ethical standards in research and experimentation.