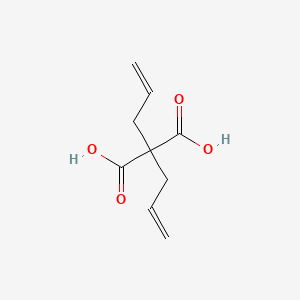
Diallylmalonic acid
Overview
Description
Scientific Research Applications
Diallylmalonic acid has a wide range of applications in scientific research:
Chemistry: It is used as a building block in organic synthesis, particularly in the preparation of complex molecules and polymers.
Biology: Its derivatives are studied for their potential biological activities, including antimicrobial and anticancer properties.
Medicine: Research is ongoing to explore its use in drug development and as a precursor for pharmaceuticals.
Industry: It is used in the production of specialty chemicals, resins, and coatings.
Safety and Hazards
Preparation Methods
Synthetic Routes and Reaction Conditions: Diallylmalonic acid can be synthesized through the alkylation of malonic acid derivatives. One common method involves the reaction of diethyl malonate with allyl bromide in the presence of a strong base such as sodium ethoxide. The reaction proceeds via a nucleophilic substitution mechanism, resulting in the formation of this compound diethyl ester, which can then be hydrolyzed to yield this compound.
Industrial Production Methods: Industrial production of this compound typically follows similar synthetic routes but on a larger scale. The process involves careful control of reaction conditions, such as temperature and pH, to ensure high yield and purity of the final product. The use of continuous flow reactors and automated systems can enhance the efficiency and scalability of the production process.
Chemical Reactions Analysis
Types of Reactions: Diallylmalonic acid undergoes various chemical reactions, including:
Oxidation: It can be oxidized to form corresponding carboxylic acids.
Reduction: Reduction reactions can convert it into alcohols or other reduced forms.
Substitution: The allyl groups can participate in substitution reactions, leading to the formation of new compounds.
Common Reagents and Conditions:
Oxidation: Common oxidizing agents include potassium permanganate and chromium trioxide.
Reduction: Reducing agents such as lithium aluminum hydride or sodium borohydride are often used.
Substitution: Reagents like halogens or nucleophiles can be used under appropriate conditions.
Major Products: The major products formed from these reactions depend on the specific reagents and conditions used. For example, oxidation can yield this compound derivatives with additional carboxyl groups, while reduction can produce diallyl alcohols.
Mechanism of Action
The mechanism of action of diallylmalonic acid involves its ability to undergo various chemical transformations. Its molecular targets and pathways depend on the specific reactions it participates in. For example, in biological systems, its derivatives may interact with cellular components, leading to specific biological effects. The exact pathways and targets are subjects of ongoing research.
Comparison with Similar Compounds
Malonic Acid: The parent compound, which lacks the allyl groups.
Diethyl Malonate: An ester derivative used in similar synthetic applications.
Allylmalonic Acid: A related compound with one allyl group.
Uniqueness: Diallylmalonic acid is unique due to the presence of two allyl groups, which confer distinct reactivity and potential for diverse chemical transformations. This makes it a valuable compound in both research and industrial applications.
Properties
IUPAC Name |
2,2-bis(prop-2-enyl)propanedioic acid | |
---|---|---|
Source | PubChem | |
URL | https://pubchem.ncbi.nlm.nih.gov | |
Description | Data deposited in or computed by PubChem | |
InChI |
InChI=1S/C9H12O4/c1-3-5-9(6-4-2,7(10)11)8(12)13/h3-4H,1-2,5-6H2,(H,10,11)(H,12,13) | |
Source | PubChem | |
URL | https://pubchem.ncbi.nlm.nih.gov | |
Description | Data deposited in or computed by PubChem | |
InChI Key |
VSJZBUJCPNVRET-UHFFFAOYSA-N | |
Source | PubChem | |
URL | https://pubchem.ncbi.nlm.nih.gov | |
Description | Data deposited in or computed by PubChem | |
Canonical SMILES |
C=CCC(CC=C)(C(=O)O)C(=O)O | |
Source | PubChem | |
URL | https://pubchem.ncbi.nlm.nih.gov | |
Description | Data deposited in or computed by PubChem | |
Molecular Formula |
C9H12O4 | |
Source | PubChem | |
URL | https://pubchem.ncbi.nlm.nih.gov | |
Description | Data deposited in or computed by PubChem | |
DSSTOX Substance ID |
DTXSID701286826 | |
Record name | 2,2-Di-2-propen-1-ylpropanedioic acid | |
Source | EPA DSSTox | |
URL | https://comptox.epa.gov/dashboard/DTXSID701286826 | |
Description | DSSTox provides a high quality public chemistry resource for supporting improved predictive toxicology. | |
Molecular Weight |
184.19 g/mol | |
Source | PubChem | |
URL | https://pubchem.ncbi.nlm.nih.gov | |
Description | Data deposited in or computed by PubChem | |
CAS No. |
4372-31-0 | |
Record name | 2,2-Di-2-propen-1-ylpropanedioic acid | |
Source | CAS Common Chemistry | |
URL | https://commonchemistry.cas.org/detail?cas_rn=4372-31-0 | |
Description | CAS Common Chemistry is an open community resource for accessing chemical information. Nearly 500,000 chemical substances from CAS REGISTRY cover areas of community interest, including common and frequently regulated chemicals, and those relevant to high school and undergraduate chemistry classes. This chemical information, curated by our expert scientists, is provided in alignment with our mission as a division of the American Chemical Society. | |
Explanation | The data from CAS Common Chemistry is provided under a CC-BY-NC 4.0 license, unless otherwise stated. | |
Record name | 2,2-Di-2-propen-1-ylpropanedioic acid | |
Source | EPA DSSTox | |
URL | https://comptox.epa.gov/dashboard/DTXSID701286826 | |
Description | DSSTox provides a high quality public chemistry resource for supporting improved predictive toxicology. | |
Q1: What are the main applications of diallylmalonic acid in chemical research?
A1: this compound and its esters are primarily used as substrates in organic synthesis and catalysis research. One key area is olefin metathesis, where this compound and its esters serve as model substrates for evaluating the efficiency and selectivity of new catalysts. [, , ] For example, this compound’s diethyl ester (diethyl diallylmalonate, DEDAM) is frequently employed to assess ring-closing metathesis (RCM) reactions. [, ] Another application utilizes this compound’s dual allyl groups in free-radical addition-cyclization reactions to create substituted cyclopentanes. []
Q2: How does the structure of this compound influence its reactivity in olefin metathesis reactions?
A2: The presence of two terminal alkene groups (allyl groups) in this compound allows it to participate in ring-closing metathesis (RCM). [, , ] During RCM, the ruthenium-based Grubbs' catalysts react with the alkene groups, ultimately leading to the formation of a cyclic compound and the release of ethylene gas. The carboxylic acid groups in this compound can influence the solubility and reactivity depending on the reaction conditions. []
Q3: Are there ways to separate ruthenium-based catalysts from products after performing olefin metathesis with this compound derivatives?
A3: Yes, researchers have developed ruthenium catalysts bearing pH-responsive ligands for improved separation. [] By incorporating pH-responsive N-heterocyclic carbene (NHC) ligands with dimethylamino groups, the catalysts can be easily protonated by adding acid. This change in charge state significantly increases the catalyst's solubility in polar solvents like water, allowing for efficient separation from the less polar RCM products through extraction methods. []
Q4: Has this compound been used in any polymer chemistry research?
A4: Yes, this compound esters have been utilized in polymer synthesis. One example involves using modified polystyrene macromonomers with terminal allyl groups, introduced via a reaction with diethyl diallylmalonate. [] These macromonomers were then copolymerized with 4-vinylpyridine to create sequential copolymers, demonstrating their potential application in synthesizing materials with controlled structures and properties. []
Q5: What spectroscopic techniques are commonly used to characterize this compound and its derivatives?
A5: While specific spectroscopic data isn't provided in the provided abstracts, researchers often utilize techniques like nuclear magnetic resonance (NMR) spectroscopy and infrared (IR) spectroscopy. NMR can confirm the structure and purity of this compound and its derivatives by analyzing the distinct chemical shifts of protons and carbons within the molecule. IR spectroscopy helps identify functional groups like carboxylic acids and alkenes present in the compound by analyzing their characteristic vibrational frequencies. Furthermore, X-ray crystallography is frequently employed to determine the solid-state structure of novel this compound-containing metal complexes. []
Q6: Are there other interesting findings related to this compound in the provided research?
A7: An intriguing finding involves the use of polydimethylsiloxane as an "active" membrane in metathesis reactions involving this compound. [] This membrane selectively allows the passage of non-polar reactants like diallyl ethers while blocking polar molecules like the deprotonated form of this compound. This selective permeability introduces a new control element in catalyst selectivity, highlighting the importance of exploring the interplay between catalyst properties and reaction environments. []
Disclaimer and Information on In-Vitro Research Products
Please be aware that all articles and product information presented on BenchChem are intended solely for informational purposes. The products available for purchase on BenchChem are specifically designed for in-vitro studies, which are conducted outside of living organisms. In-vitro studies, derived from the Latin term "in glass," involve experiments performed in controlled laboratory settings using cells or tissues. It is important to note that these products are not categorized as medicines or drugs, and they have not received approval from the FDA for the prevention, treatment, or cure of any medical condition, ailment, or disease. We must emphasize that any form of bodily introduction of these products into humans or animals is strictly prohibited by law. It is essential to adhere to these guidelines to ensure compliance with legal and ethical standards in research and experimentation.