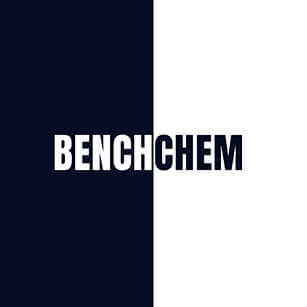
POTASSIUM HEXAFLUORONICKELATE(IV)
- Click on QUICK INQUIRY to receive a quote from our team of experts.
- With the quality product at a COMPETITIVE price, you can focus more on your research.
Overview
Description
Potassium hexafluoronickelate(IV) is an inorganic compound with the chemical formula K₂NiF₆. It is known for its strong oxidizing properties and is used in various chemical processes. The compound is typically a pink-purple crystalline powder and is highly reactive, especially with water, releasing oxygen upon contact .
Mechanism of Action
Target of Action
Potassium Hexafluoronickelate(IV) is an inorganic compound with the chemical formula
K2NiF6K_2NiF_6K2NiF6
. The primary targets of this compound are not well-documented in the literature, likely due to its inorganic nature and the complexity of its interactions with biological systems.Mode of Action
It is known to be a strong oxidant . It can turn chlorine pentafluoride and bromine pentafluoride into hexafluorochlorine cation (
ClF6+ClF_6^+ClF6+
) and hexafluorobromine cation (BrF6+BrF_6^+BrF6+
), respectively . This suggests that it may interact with its targets primarily through oxidation reactions.Result of Action
Potassium Hexafluoronickelate(IV) decomposes at high temperatures to release fluorine gas . Like terbium(IV) fluoride, the emitted fluorine is primarily monatomic rather than the typical diatomic . This suggests that its action could result in the production of reactive fluorine species, which could have various molecular and cellular effects.
Action Environment
The action of Potassium Hexafluoronickelate(IV) is influenced by environmental factors. For instance, it reacts violently with water, releasing oxygen . It also decomposes at high temperatures, releasing fluorine gas . Therefore, its action, efficacy, and stability could be significantly affected by the presence of water and the temperature of its environment.
Biochemical Analysis
Biochemical Properties
It is known to be a strong oxidant This suggests that it could potentially interact with various biomolecules, particularly those susceptible to oxidation
Cellular Effects
Given its strong oxidizing properties, it could potentially influence cell function by inducing oxidative stress . This could impact various cellular processes, including cell signaling pathways, gene expression, and cellular metabolism. These effects would need to be confirmed through experimental studies.
Molecular Mechanism
It is possible that its effects at the molecular level could be related to its strong oxidizing properties . It could potentially interact with biomolecules through oxidation, leading to changes in their function. This could include binding interactions with biomolecules, enzyme inhibition or activation, and changes in gene expression.
Temporal Effects in Laboratory Settings
It is known to decompose at high temperatures to release fluorine gas . Information on its stability, degradation, and long-term effects on cellular function observed in in vitro or in vivo studies is currently lacking.
Preparation Methods
Potassium hexafluoronickelate(IV) can be synthesized through the reaction of potassium fluoride, nickel dichloride, and fluorine. The reaction conditions require careful control to avoid violent reactions, as the compound reacts vigorously with water . Industrial production methods involve dissolving the compound in anhydrous hydrogen fluoride to produce a light-red solution .
Chemical Reactions Analysis
Potassium hexafluoronickelate(IV) undergoes several types of chemical reactions, including:
Oxidation: It acts as a strong oxidant and can oxidize other compounds.
Decomposition: At temperatures above 350°C, it decomposes to form potassium hexafluoronickelate(III), nickel(II) fluoride, and fluorine gas.
Substitution: It can react with chlorine pentafluoride and bromine pentafluoride to form ClF₆⁺ and BrF₆⁺, respectively.
Common reagents used in these reactions include chlorine pentafluoride, bromine pentafluoride, and anhydrous hydrogen fluoride. The major products formed from these reactions are fluorine gas and various nickel fluoride compounds .
Scientific Research Applications
Potassium hexafluoronickelate(IV) has several scientific research applications:
Nanomaterials: It serves as a precursor for the synthesis of nanomaterials.
Comparison with Similar Compounds
Potassium hexafluoronickelate(IV) can be compared with other similar compounds such as:
Potassium hexafluoroplatinate(IV): Similar in structure but contains platinum instead of nickel.
Potassium hexafluorotitanate(IV): Contains titanium and is used in different industrial applications.
Potassium hexafluoroantimonate(V): Contains antimony and is used as a catalyst in various chemical reactions.
Potassium hexafluoronickelate(IV) is unique due to its strong oxidizing properties and its ability to release fluorine gas upon decomposition, which is not commonly observed in similar compounds .
Properties
CAS No. |
17218-47-2 |
---|---|
Molecular Formula |
F6K2Ni+ |
Molecular Weight |
250.88 |
Origin of Product |
United States |
Q1: What makes Potassium Hexafluoronickelate(IV) a potentially valuable fluorine source?
A1: Potassium Hexafluoronickelate(IV) (K2NiF6) stands out as a promising fluorine source due to its ability to release high-purity fluorine gas upon thermal decomposition. Research demonstrates that at temperatures above 250°C, K2NiF6 decomposes, generating fluorine gas with purity exceeding 99%. [] This decomposition process even reaches a pressure of 620 kPa at 350°C, highlighting its potential for controlled fluorine gas generation. []
Q2: How is Potassium Hexafluoronickelate(IV) synthesized?
A2: Potassium Hexafluoronickelate(IV) is synthesized through a high-temperature, high-pressure reaction. A mixture of potassium fluoride (KF) and nickel fluoride (NiF2) effectively absorbs fluorine gas (F2) at temperatures exceeding 225°C and pressures around 2.0 MPa. [] This reaction leads to the formation of K2NiF6, confirmed through techniques like FT-IR and Raman spectroscopy. []
Q3: Has Potassium Hexafluoronickelate(IV) been explored for catalytic applications?
A3: Yes, Potassium Hexafluoronickelate(IV) has shown promise as a fluorinating agent in organic synthesis. Studies have explored its ability to fluorinate benzene, demonstrating its potential for facilitating halogenation reactions. [] Further research into its catalytic activity and reaction mechanisms could unveil a broader range of applications in organic synthesis.
Q4: What spectroscopic techniques provide insights into the structure of Potassium Hexafluoronickelate(IV)?
A4: Researchers have employed both infrared (IR) and Raman spectroscopy to characterize the structure of Potassium Hexafluoronickelate(IV). [, ] These techniques provide valuable information about the vibrational modes within the molecule, which helps in understanding its structure and bonding characteristics. Additionally, studies have investigated its electronic absorption spectrum to elucidate the electronic structure and transitions within the compound. []
Disclaimer and Information on In-Vitro Research Products
Please be aware that all articles and product information presented on BenchChem are intended solely for informational purposes. The products available for purchase on BenchChem are specifically designed for in-vitro studies, which are conducted outside of living organisms. In-vitro studies, derived from the Latin term "in glass," involve experiments performed in controlled laboratory settings using cells or tissues. It is important to note that these products are not categorized as medicines or drugs, and they have not received approval from the FDA for the prevention, treatment, or cure of any medical condition, ailment, or disease. We must emphasize that any form of bodily introduction of these products into humans or animals is strictly prohibited by law. It is essential to adhere to these guidelines to ensure compliance with legal and ethical standards in research and experimentation.