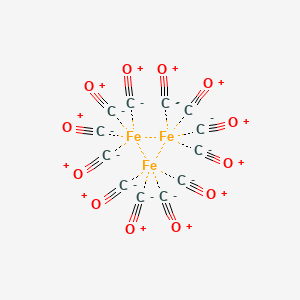
Triiron dodecacarbonyl
Overview
Description
Mechanism of Action
Triiron dodecacarbonyl, also known as Triirondodecacarbonyl or carbon monoxide;iron, is an organoiron compound with the formula Fe3(CO)12 . It is a dark green solid that sublimes under vacuum and is soluble in nonpolar organic solvents .
Target of Action
The primary targets of this compound are cyclic thioester ligands . The compound interacts with these ligands, leading to various reactions that result in the formation of different complexes .
Mode of Action
This compound reacts with cyclic thioester ligands, leading to the cleavage of the C-S bond, which is initiated by the coordination of the S atom to the Fe atom . This reaction produces a known diiron complex .
Biochemical Pathways
The reaction of cyclic thioester ligands with this compound leads to the scission of the C-S bond . The larger the ring size of the ligand, the more diverse are the bond cleavages . This suggests that the compound affects biochemical pathways involving C-S bonds.
Pharmacokinetics
It is known that the compound is a dark green solid that sublimes under vacuum . It is soluble in nonpolar organic solvents, which may influence its absorption, distribution, metabolism, and excretion (ADME) properties .
Result of Action
The result of the action of this compound is the formation of different complexes through the cleavage of C-S bonds in cyclic thioester ligands
Action Environment
The action of this compound is influenced by environmental factors. For instance, the compound sublimes under vacuum and is soluble in nonpolar organic solvents . Additionally, the compound decomposes slowly in air, and thus samples are typically stored cold under an inert atmosphere . These factors can influence the compound’s action, efficacy, and stability.
Biochemical Analysis
Biochemical Properties
Triirondodecacarbonyl is a more reactive source of iron (0) than iron pentacarbonyl
Temporal Effects in Laboratory Settings
Triirondodecacarbonyl is known to decompose to an iron mirror when hot solutions of it are prepared . The solid decomposes slowly in air, and thus samples are typically stored cold under an inert atmosphere
Preparation Methods
Triiron dodecacarbonyl was one of the first metal carbonyl clusters synthesized. It can be obtained from the thermolysis of iron pentacarbonyl (Fe(CO)₅): [ 3 \text{Fe(CO)}_5 \rightarrow \text{Fe}3(\text{CO}){12} + 3 \text{CO} ] Another common synthetic route involves the reaction of iron pentacarbonyl with a base, followed by oxidation of the resulting hydrido cluster with acid : [ 3 \text{Fe(CO)}_5 + (\text{C}_2\text{H}_5)_3\text{N} + \text{H}_2\text{O} \rightarrow [(\text{C}_2\text{H}_5)_3\text{NH}][\text{HFe}3(\text{CO}){11}] + 3 \text{CO} + \text{CO}_2 ] [ [(\text{C}_2\text{H}_5)_3\text{NH}][\text{HFe}3(\text{CO}){11}] + \text{HCl} + \text{CO} \rightarrow \text{Fe}3(\text{CO}){12} + \text{H}_2 + [(\text{C}_2\text{H}_5)_3\text{NH}]\text{Cl} ]
Chemical Reactions Analysis
Triiron dodecacarbonyl undergoes various types of chemical reactions, including:
Oxidation: It can be oxidized to form different iron carbonyl complexes.
Reduction: The compound can be reduced under specific conditions to yield other iron carbonyl species.
Substitution: Ligands in triirondodecacarbonyl can be substituted with other ligands such as phosphines or thioesters. Common reagents used in these reactions include bases like triethylamine, acids like hydrochloric acid, and various ligands for substitution reactions.
Scientific Research Applications
Triiron dodecacarbonyl has several scientific research applications:
Comparison with Similar Compounds
Triiron dodecacarbonyl can be compared with other similar compounds such as:
Triruthenium dodecacarbonyl (Ru₃(CO)₁₂): Similar in structure but contains ruthenium instead of iron.
Triosmium dodecacarbonyl (Os₃(CO)₁₂): Another analogous compound with osmium atoms.
Iron pentacarbonyl (Fe(CO)₅): A simpler iron carbonyl compound with five CO ligands. This compound is unique due to its triangular structure and the specific reactivity of its iron atoms, making it a valuable compound in various chemical applications.
Biological Activity
Triiron dodecacarbonyl, with the chemical formula Fe(CO), is an organometallic compound that has garnered interest in various fields, particularly in bioinorganic chemistry due to its structural resemblance to metalloenzymes. This article explores its biological activity, focusing on its mechanisms of action, potential applications, and relevant research findings.
This compound is a dark green solid that sublimates under vacuum and is soluble in nonpolar organic solvents, producing intensely green solutions. Its molecular structure features a triangular arrangement of iron atoms coordinated by twelve carbon monoxide ligands, which play a crucial role in its reactivity and biological interactions .
The biological activity of this compound is primarily attributed to its ability to mimic the active sites of certain metalloenzymes. The compound's iron atoms can coordinate with various ligands, enabling it to facilitate reactions similar to those catalyzed by natural enzymes. The triangular geometry allows for unique interactions that can lead to ligand substitution and bond cleavage, which are essential for catalytic processes .
Biological Applications
1. Enzyme Model Studies:
this compound has been utilized as a model compound for studying hydrogenase enzymes. Research indicates that it can effectively catalyze proton reduction reactions, making it a valuable tool for investigating enzyme mechanisms and developing bioinspired catalysts .
2. Toxicological Studies:
Despite its potential applications, this compound poses significant toxicity risks. It can carbonylate hemoglobin, leading to the formation of carboxyhemoglobin, which inhibits oxygen transport in the blood. This property necessitates careful handling and consideration of safety protocols when working with this compound .
Case Studies
- Catalytic Activity: A study demonstrated that this compound could serve as a precursor for synthesizing iron-sulfur clusters that exhibit catalytic activity for proton reduction at low overpotentials. This finding highlights the compound's potential in developing efficient catalysts for energy conversion applications .
- Structural Analysis: X-ray diffraction studies have provided insights into the structural characteristics of this compound and its derivatives. These studies reveal how modifications to the ligand environment can influence the electronic properties and reactivity of the iron core .
Data Table: Summary of Biological Activity Research
Properties
IUPAC Name |
carbon monoxide;iron | |
---|---|---|
Source | PubChem | |
URL | https://pubchem.ncbi.nlm.nih.gov | |
Description | Data deposited in or computed by PubChem | |
InChI |
InChI=1S/12CO.3Fe/c12*1-2;;; | |
Source | PubChem | |
URL | https://pubchem.ncbi.nlm.nih.gov | |
Description | Data deposited in or computed by PubChem | |
InChI Key |
HLYRMDDXFDINCB-UHFFFAOYSA-N | |
Source | PubChem | |
URL | https://pubchem.ncbi.nlm.nih.gov | |
Description | Data deposited in or computed by PubChem | |
Canonical SMILES |
[C-]#[O+].[C-]#[O+].[C-]#[O+].[C-]#[O+].[C-]#[O+].[C-]#[O+].[C-]#[O+].[C-]#[O+].[C-]#[O+].[C-]#[O+].[C-]#[O+].[C-]#[O+].[Fe].[Fe].[Fe] | |
Source | PubChem | |
URL | https://pubchem.ncbi.nlm.nih.gov | |
Description | Data deposited in or computed by PubChem | |
Molecular Formula |
C12Fe3O12 | |
Source | PubChem | |
URL | https://pubchem.ncbi.nlm.nih.gov | |
Description | Data deposited in or computed by PubChem | |
Molecular Weight |
503.65 g/mol | |
Source | PubChem | |
URL | https://pubchem.ncbi.nlm.nih.gov | |
Description | Data deposited in or computed by PubChem | |
CAS No. |
17685-52-8 | |
Record name | Dodecacarbonyl iron | |
Source | ChemIDplus | |
URL | https://pubchem.ncbi.nlm.nih.gov/substance/?source=chemidplus&sourceid=0017685528 | |
Description | ChemIDplus is a free, web search system that provides access to the structure and nomenclature authority files used for the identification of chemical substances cited in National Library of Medicine (NLM) databases, including the TOXNET system. | |
Q1: What is the molecular formula and weight of Triirondodecacarbonyl?
A1: The molecular formula of Triirondodecacarbonyl is Fe₃(CO)₁₂. Its molecular weight is 391.85 g/mol.
Q2: How can spectroscopic data aid in characterizing Triirondodecacarbonyl?
A2: Spectroscopic techniques like Infrared (IR) spectroscopy and UV-vis spectroscopy offer valuable insights into the structure and bonding of Fe₃(CO)₁₂. [] IR spectroscopy identifies characteristic stretching frequencies of carbonyl (CO) ligands, providing information about the coordination environment of the iron atoms. [, , ] UV-vis spectroscopy reveals electronic transitions within the molecule, offering insights into its electronic structure and reactivity. []
Q3: Can quantum chemical simulations provide information about Triirondodecacarbonyl?
A3: Yes, quantum chemical simulations can be used to investigate the structure and reactivity of Fe₃(CO)₁₂. [] For instance, these simulations can be used to predict the vibrational frequencies of the molecule, which can be compared to experimental IR spectra. [] They can also be used to study the mechanism of reactions involving Fe₃(CO)₁₂. []
Q4: What are the typical reactions Triirondodecacarbonyl participates in?
A4: Fe₃(CO)₁₂ readily undergoes reactions with various ligands, including thiols, disulfides, and selenium or tellurium analogues, to form iron-sulfur cluster complexes. [] It is also known to participate in metal exchange reactions, as observed in its reaction with cyclooctatetraenechromiumtricarbonyl. []
Q5: How does N-methyl-2-pyrrolidone (NMP) influence reactions involving Triirondodecacarbonyl?
A5: NMP acts as an aprotic polar co-solvent and significantly enhances the reaction rate of Fe₃(CO)₁₂ with various ligands. [] Kinetic studies using UV-vis spectroscopy show a tenfold increase in the rate constant for the formation of certain [FeFe] hydrogenase mimics when NMP is present. []
Q6: What is the proposed mechanism for the NMP-mediated enhancement of Triirondodecacarbonyl reactivity?
A6: Research suggests that NMP interacts with Fe₃(CO)₁₂, likely forming a monosubstituted triirondodecacarbonyl (Fe3(CO)11NMP) cluster. [] IR spectroscopy analysis supports the formation of this intermediate. [] Quantum chemical simulations indicate that this interaction disrupts the bridging carbonyl groups (μ-CO) in Fe₃(CO)₁₂, potentially enhancing its reactivity. []
Q7: Does Triirondodecacarbonyl exhibit catalytic activity?
A7: Yes, Fe₃(CO)₁₂ demonstrates catalytic activity in various organic reactions. One notable example is its ability, in combination with indium trichloride (InCl3), to catalyze the selective double hydrosilylation of nitriles using tertiary and secondary silanes. []
Q8: Are there other examples of Triirondodecacarbonyl’s catalytic activity?
A8: Yes, Fe₃(CO)₁₂ can be used to synthesize specific organic compounds. For example, it facilitates the synthesis of methyl 7-oxoheptanoate and methyl 7-oxooctanoate through a nucleophilic acylation reaction with disodium tetracarbonylferrate. [] This reaction involves the use of Fe₃(CO)₁₂ as a starting material to generate the reactive disodium tetracarbonylferrate intermediate. []
Q9: What are the potential applications of Triirondodecacarbonyl and its derivatives?
A9: Fe₃(CO)₁₂ and its derivatives hold promise for various applications. Their ability to form iron-sulfur clusters makes them valuable in mimicking the active sites of [FeFe] hydrogenases, enzymes crucial for hydrogen production and utilization. [] Additionally, their catalytic activity opens avenues for new synthetic routes in organic chemistry. []
Q10: What are the challenges associated with using Triirondodecacarbonyl in practical applications?
A10: One challenge is the sensitivity of Fe₃(CO)₁₂ to air and moisture, which necessitates handling under inert conditions. [, ] Additionally, optimizing reaction conditions and understanding the detailed mechanisms of its reactions are crucial for developing efficient and selective catalytic processes.
Q11: What safety precautions should be taken when handling Triirondodecacarbonyl?
A11: Fe₃(CO)₁₂ should be handled with care as it decomposes into iron and carbon monoxide, a toxic gas, upon heating or exposure to air. [] Appropriate laboratory practices, including the use of a fume hood and personal protective equipment, are crucial when working with this compound. []
Disclaimer and Information on In-Vitro Research Products
Please be aware that all articles and product information presented on BenchChem are intended solely for informational purposes. The products available for purchase on BenchChem are specifically designed for in-vitro studies, which are conducted outside of living organisms. In-vitro studies, derived from the Latin term "in glass," involve experiments performed in controlled laboratory settings using cells or tissues. It is important to note that these products are not categorized as medicines or drugs, and they have not received approval from the FDA for the prevention, treatment, or cure of any medical condition, ailment, or disease. We must emphasize that any form of bodily introduction of these products into humans or animals is strictly prohibited by law. It is essential to adhere to these guidelines to ensure compliance with legal and ethical standards in research and experimentation.