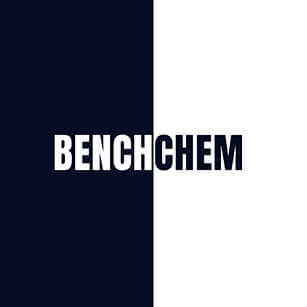
MOLYBDENUM PHTHALOCYANINE
- Click on QUICK INQUIRY to receive a quote from our team of experts.
- With the quality product at a COMPETITIVE price, you can focus more on your research.
Overview
Description
Molybdenum phthalocyanine is a coordination compound that belongs to the phthalocyanine family, which are macrocyclic compounds known for their vivid blue or green colors. These compounds have been widely studied due to their excellent optical and electrochemical properties.
Mechanism of Action
Target of Action
Molybdenum phthalocyanine primarily targets cellular components involved in oxidative stress and electron transport. Its primary role is to act as a photosensitizer in photodynamic therapy (PDT), where it targets tumor cells by generating reactive oxygen species (ROS) upon light activation .
Mode of Action
Upon light activation, this compound absorbs photons and transitions to an excited state. This excited state interacts with molecular oxygen to produce singlet oxygen and other ROS. These reactive species cause oxidative damage to cellular components, leading to cell death, particularly in cancer cells .
Biochemical Pathways
The primary biochemical pathway affected by this compound is the oxidative stress pathway. The generated ROS can damage lipids, proteins, and DNA, leading to apoptosis or necrosis of the targeted cells. This disruption in cellular homeostasis triggers downstream effects such as activation of caspases and other apoptotic pathways .
Pharmacokinetics
The pharmacokinetics of this compound involve its absorption, distribution, metabolism, and excretion (ADME). It is typically administered intravenously, allowing for rapid distribution to tissues. Its bioavailability is influenced by its solubility and stability in biological environments. Metabolism primarily occurs in the liver, and excretion is through the renal and biliary systems .
Result of Action
The molecular and cellular effects of this compound’s action include the induction of oxidative stress, leading to cell membrane damage, mitochondrial dysfunction, and DNA fragmentation. These effects culminate in the death of cancer cells, making it an effective agent in photodynamic therapy .
Action Environment
Environmental factors such as light intensity, oxygen availability, and the presence of antioxidants can influence the efficacy and stability of this compound. Optimal light conditions are crucial for its activation, while sufficient oxygen levels are necessary for ROS generation. Antioxidants in the environment can mitigate the compound’s effectiveness by neutralizing ROS .
Biochemical Analysis
Biochemical Properties
Molybdenum Phthalocyanine plays a crucial role in biochemical reactions, particularly in redox processes. It interacts with various enzymes, proteins, and other biomolecules, facilitating electron transfer reactions. For instance, this compound can interact with cytochrome P450 enzymes, enhancing their catalytic activity in the oxidation of organic substrates . Additionally, it has been shown to interact with superoxide dismutase, contributing to its antioxidant properties . These interactions are primarily driven by the ability of this compound to undergo reversible redox changes, making it an effective mediator in biochemical processes.
Cellular Effects
This compound exerts various effects on different cell types and cellular processes. It influences cell function by modulating cell signaling pathways, gene expression, and cellular metabolism. For example, this compound has been observed to activate the mitogen-activated protein kinase (MAPK) pathway, leading to changes in gene expression that promote cell proliferation and survival . Furthermore, it can affect cellular metabolism by altering the activity of key metabolic enzymes, thereby impacting energy production and biosynthetic pathways .
Molecular Mechanism
The molecular mechanism of action of this compound involves its ability to bind to biomolecules and modulate their activity. It can act as an enzyme inhibitor or activator, depending on the specific target. For instance, this compound has been shown to inhibit the activity of xanthine oxidase, reducing the production of reactive oxygen species . Additionally, it can activate certain transcription factors, leading to changes in gene expression that influence cellular responses . These effects are mediated through the binding interactions of this compound with specific biomolecules, which alter their conformation and activity.
Temporal Effects in Laboratory Settings
In laboratory settings, the effects of this compound can change over time due to its stability, degradation, and long-term impact on cellular function. Studies have shown that this compound is relatively stable under physiological conditions, maintaining its activity over extended periods . Prolonged exposure to light or oxidative stress can lead to its degradation, resulting in reduced efficacy . Long-term studies have also indicated that this compound can induce adaptive responses in cells, such as upregulation of antioxidant defenses .
Dosage Effects in Animal Models
The effects of this compound vary with different dosages in animal models. At low doses, it has been shown to enhance cellular functions and promote tissue repair . At high doses, this compound can exhibit toxic effects, including oxidative stress, inflammation, and tissue damage . Threshold effects have been observed, where the beneficial effects are maximized at optimal dosages, while adverse effects become prominent at higher concentrations .
Metabolic Pathways
This compound is involved in various metabolic pathways, interacting with enzymes and cofactors that regulate metabolic flux. It can modulate the activity of enzymes such as aldehyde oxidase and sulfite oxidase, influencing the metabolism of aldehydes and sulfites . Additionally, this compound can affect the levels of metabolites by altering the balance between anabolic and catabolic processes . These interactions highlight the role of this compound in maintaining metabolic homeostasis.
Transport and Distribution
Within cells and tissues, this compound is transported and distributed through interactions with transporters and binding proteins. It can bind to albumin and other plasma proteins, facilitating its transport in the bloodstream . Additionally, this compound can be taken up by cells through endocytosis and distributed to various cellular compartments . Its localization and accumulation within specific tissues are influenced by factors such as tissue perfusion and cellular uptake mechanisms .
Subcellular Localization
The subcellular localization of this compound plays a critical role in its activity and function. It can be targeted to specific compartments or organelles through targeting signals or post-translational modifications . For example, this compound has been observed to localize to the mitochondria, where it can modulate mitochondrial function and energy production . Additionally, its presence in the nucleus can influence gene expression by interacting with nuclear proteins and transcription factors .
Preparation Methods
Synthetic Routes and Reaction Conditions: Molybdenum phthalocyanine can be synthesized through the cyclotetramerization of phthalonitrile derivatives in the presence of molybdenum salts. One common method involves the reaction of phthalonitrile with molybdenum chloride in a high-boiling solvent such as quinoline at elevated temperatures (around 200-300°C). The reaction typically requires a nitrogen atmosphere to prevent oxidation .
Industrial Production Methods: Industrial production of this compound follows similar synthetic routes but on a larger scale. The process involves the use of large reactors and precise control of reaction conditions to ensure high yield and purity. The product is then purified through recrystallization or chromatography techniques to remove any impurities .
Chemical Reactions Analysis
Types of Reactions: Molybdenum phthalocyanine undergoes various chemical reactions, including:
Oxidation: It can be oxidized to form oxo-molybdenum phthalocyanine complexes.
Reduction: Reduction reactions can lead to the formation of reduced this compound species.
Common Reagents and Conditions:
Oxidation: Common oxidizing agents include hydrogen peroxide and oxygen.
Reduction: Reducing agents such as sodium borohydride or hydrazine are often used.
Substitution: Substitution reactions typically involve nucleophiles such as amines or thiols under mild conditions.
Major Products Formed:
Oxidation: Oxo-molybdenum phthalocyanine complexes.
Reduction: Reduced this compound species.
Substitution: Functionalized this compound derivatives.
Scientific Research Applications
Molybdenum phthalocyanine has a wide range of scientific research applications, including:
Comparison with Similar Compounds
- Iron Phthalocyanine
- Cobalt Phthalocyanine
- Nickel Phthalocyanine
- Copper Phthalocyanine
Comparison: Molybdenum phthalocyanine is unique due to its central molybdenum atom, which imparts distinct catalytic and electronic properties compared to other metal phthalocyanines. For instance, this compound exhibits higher catalytic activity in certain oxidation reactions compared to iron or cobalt phthalocyanine . Additionally, its ability to generate reactive oxygen species upon light activation makes it particularly suitable for photodynamic therapy applications .
Properties
CAS No. |
15152-82-6 |
---|---|
Molecular Formula |
C32H16MoN8 |
Molecular Weight |
608.46 |
Origin of Product |
United States |
Q1: What makes Molybdenum phthalocyanine (Mo-Pc) a promising candidate for producing high specific activity Molybdenum-99 (Mo-99)?
A1: Mo-Pc shows promise due to its suitability for the Szilard-Chalmers effect, a recoil reaction triggered by neutron irradiation [, ]. This process can produce Mo-99 with significantly higher specific activity than traditional methods using Molybdenum trioxide (MoO3) targets []. This is crucial for developing smaller, more efficient radioisotope generators for medical applications [].
Q2: How is Mo-Pc synthesized, and what are its key structural characteristics?
A2: Mo-Pc can be synthesized through a solid-state reaction between ammonium heptamolybdate and phthalonitrile at high temperatures (around 300°C) []. The resulting compound, Phthalocyanine–oxymolybdenum(IV) with the molecular formula MoOC32H16N8, is a stable, diamagnetic complex [].
Q3: Can you elaborate on the spectroscopic properties of Mo-Pc?
A3: Mo-Pc exhibits characteristic Q-band absorption in the UV-Vis spectrum between 600 and 750 nm, depending on the solvent used []. Splitting of the absorption peak in solvents like tetrahydrofuran and dimethylformamide suggests protonation of the molecule [].
Q4: What is the significance of optimizing the extraction process for Mo-99 from irradiated Mo-Pc?
A4: Efficient extraction of Mo-99 from the irradiated Mo-Pc target is crucial for maximizing yield and achieving high specific activity []. Studies have investigated various solvents and extraction parameters to optimize this process and determine the ideal conditions for high yield and purity of the Mo-99 product [].
Q5: What are the potential applications of Mo-Pc beyond radioisotope production?
A5: Research suggests Mo-Pc displays rectification properties when sandwiched between different metal electrodes, particularly in the presence of polar impurities []. This characteristic hints at its potential use in electronic devices. Additionally, studies have explored the electrochromic behavior of Mo-Pc oxide, suggesting possible applications in display technologies [].
Disclaimer and Information on In-Vitro Research Products
Please be aware that all articles and product information presented on BenchChem are intended solely for informational purposes. The products available for purchase on BenchChem are specifically designed for in-vitro studies, which are conducted outside of living organisms. In-vitro studies, derived from the Latin term "in glass," involve experiments performed in controlled laboratory settings using cells or tissues. It is important to note that these products are not categorized as medicines or drugs, and they have not received approval from the FDA for the prevention, treatment, or cure of any medical condition, ailment, or disease. We must emphasize that any form of bodily introduction of these products into humans or animals is strictly prohibited by law. It is essential to adhere to these guidelines to ensure compliance with legal and ethical standards in research and experimentation.