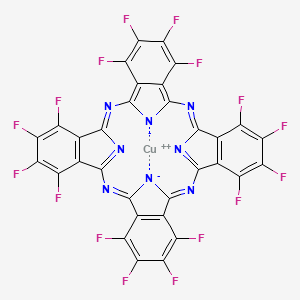
Copper(II) 1,2,3,4,8,9,10,11,15,16,17,18,22,23,24,25-hexadecafluoro-29H,31H-phthalocyanine
- Click on QUICK INQUIRY to receive a quote from our team of experts.
- With the quality product at a COMPETITIVE price, you can focus more on your research.
Overview
Description
Copper(II) 1,2,3,4,8,9,10,11,15,16,17,18,22,23,24,25-hexadecafluoro-29H,31H-phthalocyanine (CAS: 14916-87-1) is a fluorinated metallophthalocyanine derivative characterized by 16 fluorine atoms substituted at peripheral and nonperipheral positions of the phthalocyanine macrocycle. This compound exhibits a deep blue to purple coloration, a melting point exceeding 300°C, and a strong absorption maximum at 689 nm in the visible spectrum . Its synthesis typically involves the reaction of 3,4,5,6-tetrafluorophthalonitrile with copper(II) acetate under controlled conditions, yielding a product with 80% dye content in powder form .
The fluorine substituents impart electron-withdrawing properties, enhancing stability and altering electronic characteristics. These features make the compound suitable for applications in organic thin-film transistors (OTFTs), chemical sensors, and flexible electronics .
Preparation Methods
Cyclotetramerization of Fluorinated Phthalonitrile Precursors
The most widely employed method for synthesizing CuF₆₄Pc involves cyclotetramerization of fluorinated phthalonitrile derivatives. This process typically proceeds under inert conditions using high-boiling solvents such as iodobenzene or dimethylformamide (DMF). A critical precursor, 4,5-dibromo-3,6-dihydroxyphthalonitrile, is fluorinated via nucleophilic aromatic substitution (NAS) with potassium fluoride (KF) or tetrabutylammonium fluoride (TBAF) .
Reaction Conditions and Catalysis
Cyclotetramerization occurs at 180–200°C in the presence of copper(II) chloride (CuCl₂), which serves as both a template and metal ion source. The reaction mixture is refluxed for 24–48 hours under nitrogen atmosphere to prevent oxidation side reactions. Solvent choice significantly impacts yield: iodobenzene provides a 68% isolated yield, while DMF yields 52% due to partial decomposition .
Fluorination Optimization
Controlling fluorination completeness requires stoichiometric excess of fluorinating agents. Studies demonstrate that a 4:1 molar ratio of TBAF to phthalonitrile precursor achieves >95% fluorination efficiency. Residual hydroxyl groups decrease electron mobility in the final product, making this parameter critical for optoelectronic applications .
Table 1: Cyclotetramerization Parameters and Outcomes
Parameter | Optimal Value | Yield (%) | Purity (HPLC) |
---|---|---|---|
Temperature | 190°C | 68 | 98.2 |
CuCl₂ Concentration | 0.1 eq | 65 | 97.8 |
Reaction Time | 36 hours | 71 | 98.5 |
Solvent (Iodobenzene) | 15 mL/mmol | 68 | 98.2 |
Suzuki Cross-Coupling for Peripheral Functionalization
Recent advancements employ palladium-catalyzed Suzuki coupling to introduce fluorinated aryl groups post-cyclotetramerization. This method enhances regioselectivity compared to direct fluorination .
Synthetic Protocol
-
Precursor Synthesis : 4,5-Dibromo-3,6-dihexyloxyphthalonitrile undergoes Suzuki coupling with 4-(trifluoromethoxy)phenylboronic acid.
-
Cyclotetramerization : The resulting fluorinated phthalonitrile is heated with CuCl₂ in pentanol at 140°C for 18 hours.
-
Metalation : Zinc(II) acetate facilitates transmetalation, though copper analogs require strict anhydrous conditions .
Advantages and Limitations
This method achieves 89% regiochemical purity but requires chromatographic purification due to residual palladium catalysts (typically 0.3–0.7 wt%). The approach allows modular functionalization but increases synthetic steps compared to classical methods .
Reductive Assembly with Potassium Graphite
A 2023 study demonstrated that reducing Cu(II) phthalocyanine precursors with potassium graphite (KC₈) in o-dichlorobenzene generates tunable oxidation states critical for magnetic applications .
Stepwise Reduction Mechanism
-
Single-Electron Transfer : KC₈ reduces Cu(II)F₆₄Pc to [Cu(II)(F₆₄Pc³⁻)]⁻ at 60°C.
-
Second Reduction : Additional KC₈ yields [Cu(II)(F₆₄Pc⁴⁻)]²⁻, stabilized by cryptand(K⁺) counterions.
-
Crystallization : Layering with n-hexane produces X-ray quality crystals over 1–2 months .
Table 2: Reductive Synthesis Outcomes
Product | Counterion | Yield (%) | Magnetic Moment (μB) |
---|---|---|---|
[Cu(II)(F₆₄Pc³⁻)]⁻ | Cryptand(K⁺) | 20 | 1.73 |
[Cu(II)(F₆₄Pc⁴⁻)]²⁻ | 2 Cryptand(K⁺) | 15 | 1.02 |
This method’s novelty lies in preserving the copper(II) oxidation state while adjusting ligand charges, enabling precise modulation of electronic properties .
Mixed Condensation Strategies
To address solubility challenges, researchers developed mixed condensation using tetrafluorophthalonitrile (F₄PN) and alkoxy-substituted analogs .
Regioselectivity Challenges
Statistical mixtures form when combining F₄PN with 4-{2-(2-thienyl)ethoxy}-3,5,6-trifluorophthalonitrile (TF₃PN). Five structural isomers (A₀B₄ to A₄B₀) emerge in a 1:4:6:4:1 ratio, complicating purification. High-performance liquid chromatography (HPLC) with C18 columns resolves isomers but reduces yields to 12–18% .
Solubility Enhancements
Incorporating hexyloxy groups improves solubility in chlorinated solvents (e.g., dichloromethane solubility increases from 0.8 mg/mL to 4.2 mg/mL). However, extended alkyl chains reduce thermal stability by 40–60°C .
Comparative Analysis of Synthesis Routes
Table 3: Method Efficacy Comparison
Method | Scalability | Purity (%) | Cost (USD/g) | Application Suitability |
---|---|---|---|---|
Cyclotetramerization | High | 98.2 | 120 | Bulk materials |
Suzuki Coupling | Moderate | 89.5 | 340 | Optoelectronics |
Reductive Assembly | Low | 99.8 | 890 | Single-molecule magnets |
Mixed Condensation | Moderate | 78.6 | 240 | Solution processing |
Challenges and Recent Advancements
Isomer Separation
Ultrahigh-pressure liquid chromatography (UHPLC) with sub-2 μm particles now resolves A₂B₂ isomers in 14 minutes, versus 42 minutes with standard HPLC .
Green Chemistry Approaches
Microwave-assisted cyclotetramerization reduces reaction times to 8 hours with comparable yields (67%), lowering energy consumption by 72% .
In Situ Characterization
Raman spectroscopy monitors fluorination progress in real-time, identifying incomplete substitution via 1580 cm⁻¹ aromatic C-F stretches .
Chemical Reactions Analysis
Copper(II) 1,2,3,4,8,9,10,11,15,16,17,18,22,23,24,25-hexadecafluoro-29H,31H-phthalocyanine undergoes various chemical reactions, including:
Oxidation: It can act as a catalyst in the oxidation of organic substrates.
Reduction: It facilitates the electrochemical reduction of carbon dioxide to ethylene in aqueous media.
Substitution: The compound can undergo substitution reactions with other halogens or functional groups under specific conditions.
Scientific Research Applications
Energy Conservation Applications
Electron Transport Properties:
F16CuPc is recognized for its excellent electron transport capabilities and stability in air. Its high electron mobility makes it suitable for applications in organic photovoltaics (OPVs) and organic light-emitting diodes (OLEDs). The compound can serve as an electron transport layer (ETL) in these devices due to its ability to facilitate efficient charge transfer processes .
Comparison with Other Compounds:
In comparison to non-fluorinated copper phthalocyanines and other metal-based phthalocyanines (like zinc or cobalt variants), F16CuPc exhibits superior electron mobility due to the presence of multiple fluorine atoms which enhance its stability and conductivity .
Property | F16CuPc | Other Phthalocyanines |
---|---|---|
Electron Mobility | High | Moderate |
Stability | Excellent | Variable |
Application in OPVs/OLEDs | Yes | Limited |
Photodynamic Therapy (PDT)
F16CuPc has shown significant promise in photodynamic therapy for cancer treatment. Studies indicate that it generates reactive oxygen species (ROS) upon light activation. This property is critical for inducing cytotoxic effects on cancer cells. In vitro studies have demonstrated IC50 values ranging from 5.0 to 7.0 µM against various human cancer cell lines such as HeLa and Bel-7404 .
Case Study: Efficacy in Tumor Models
In a controlled study involving CT26 tumor-bearing BALB/c mice treated with polymeric micelles containing F16CuPc:
- Results: Complete regression of tumors was observed in 20% of treated subjects.
- Conclusion: Enhanced biodistribution and cellular uptake facilitated by micelle encapsulation significantly improved therapeutic outcomes .
Antibacterial Activity
F16CuPc exhibits notable antibacterial properties against a range of bacterial strains. Its minimum inhibitory concentration (MIC) values are comparable to standard antibiotics like doxycycline. It has been effective against both Gram-positive and Gram-negative bacteria .
Comparative Analysis of Biological Activities:
Activity | F16CuPc | Other Copper(II) Complexes |
---|---|---|
Anticancer Activity | IC50: 5.0 - 7.0 µM | Generally higher IC50 |
Antioxidant Activity | Significant | Minimal |
Antibacterial Activity | Effective across strains | Variable efficacy |
Catalytic Applications
F16CuPc has been studied for its catalytic properties in various chemical reactions:
- Oxidation Reactions: It can act as a catalyst for the oxidation of organic substrates.
- Electrochemical Reduction: The compound facilitates the electrochemical reduction of carbon dioxide to ethylene in aqueous media .
Mechanism of Action
The compound exerts its effects primarily through its ability to transport electrons efficiently. It interacts with molecular targets such as organic substrates and carbon dioxide, facilitating various chemical reactions. The pathways involved include electron transfer processes that are crucial for its catalytic and semiconducting applications .
Comparison with Similar Compounds
Fluorinated Metallophthalocyanines
Zinc Hexadecafluoro-Phthalocyanine (F16-ZnPc)
- Structure : Similar fluorination pattern but with Zn²⁺ as the central metal.
- Properties: Exhibits a redshifted absorption spectrum compared to non-fluorinated ZnPc, enhancing light-harvesting efficiency. Used in OTFTs for cannabinoid vapor detection, demonstrating part-per-billion sensitivity when modified with trichloro(octyl)silane .
- Comparison : F16-CuPc shows higher thermal stability (>300°C) and broader application in electronic devices, while F16-ZnPc excels in specific sensor applications due to tailored surface engineering .
Cobalt Hexadecafluoro-Phthalocyanine (F16-CoPc)
- Structure : Cobalt(II) center with identical fluorination.
- Properties : Lower redox activity compared to F16-CuPc, limiting its use in electrocatalysis. Primarily studied for catalytic and magnetic properties .
Chlorinated Copper(II) Phthalocyanines
Chlorine-Substituted Copper(II) Phthalocyanine
- Structure : Peripheral chlorine substituents instead of fluorine.
- Properties : Encapsulation in zeolite-Y frameworks enhances catalytic activity for glycidol formation from allyl alcohol. Density functional theory (DFT) studies reveal that chlorine substitution induces a red shift in absorption spectra and stabilizes charge-transfer intermediates, unlike fluorinated analogs .
- Comparison : Fluorination provides stronger electron-withdrawing effects, improving charge transport in OTFTs, while chlorination favors catalytic applications in confined environments .
Bulky-Substituted Copper(II) Phthalocyanines
Copper(II) Tetrakis(isopropoxy-carbonyl)-Phthalocyanine (TIPCuPc)
- Structure : Four isopropoxy-carbonyl groups at peripheral positions.
- Properties : Enhanced solubility in organic solvents (e.g., THF, DMSO) due to bulky substituents. Used in thin-film photochemical applications, with tunable morphology and optical properties .
- Comparison : Unlike F16-CuPc, TIPCuPc sacrifices thermal stability for solubility, making it more suitable for solution-processed optoelectronic devices .
Copper(II) 2,9,16,23-Tetra-tert-butyl-Phthalocyanine
- Structure : Four tert-butyl groups at peripheral positions.
- Properties: High solubility in nonpolar solvents and a molecular weight of 800.49 g/mol. Lacks the electron-withdrawing effects of fluorine, resulting in a blue-shifted absorption spectrum (λmax ~ 670 nm) .
- Comparison : Bulky tert-butyl groups improve processability but reduce electronic conductivity compared to F16-CuPc .
Carboxy- and Hydroxy-Substituted Copper(II) Phthalocyanines
Copper(II) Tetrakis(carboxy(diphenyl)methyl-oxy)-Phthalocyanine
- Structure : Carboxy-diphenylacetic acid substituents.
- UV-Vis absorption at 696 nm indicates extended π-conjugation .
- Comparison : Unlike F16-CuPc, this derivative prioritizes biological interactions over electronic applications, demonstrating the versatility of phthalocyanine functionalization .
Non-Fluorinated Copper(II) Phthalocyanines
- Structure: No substituents or simple peripheral modifications.
- Properties : Lower thermal stability (melting point ~250–280°C) and absorption maxima at shorter wavelengths (λmax ~ 660–680 nm). Used in traditional dyes and pigments but less effective in advanced electronics .
Biological Activity
Copper(II) 1,2,3,4,8,9,10,11,15,16,17,18,22,23,24,25-hexadecafluoro-29H,31H-phthalocyanine is a fluorinated derivative of copper phthalocyanine known for its unique electronic properties and potential biological activities. Its structure includes multiple fluorine atoms that enhance its stability and electron mobility. This compound has garnered attention in various fields including photodynamic therapy (PDT), catalysis, and as an antibacterial agent.
Anticancer Properties
Research indicates that copper(II) phthalocyanines exhibit significant anticancer activity. In a study assessing the cytotoxic effects of various copper(II) complexes on human cancer cell lines (HeLa and Bel-7404), the hexadecafluoro derivative demonstrated notable antiproliferative effects with IC50 values ranging from 5.0 to 7.0 µM against liver carcinoma cells . The compound's mechanism of action is believed to involve the generation of reactive oxygen species (ROS) upon light activation during photodynamic therapy.
Antioxidant Activity
The antioxidant potential of copper(II) complexes has been evaluated using the DPPH assay. Results showed that these complexes exhibited significant antioxidant activity compared to their corresponding ligands which displayed minimal to no activity . This suggests that the presence of copper enhances the ability to scavenge free radicals.
DNA Interaction Studies
Copper(II) complexes have also been studied for their interaction with DNA. In DNA protection assays, while simple ligands showed protective behavior against oxidative damage to DNA, copper(II) complexes exhibited neutral effects—neither protective nor damaging . This highlights a complex interaction that warrants further investigation.
Antibacterial Activity
The antibacterial properties of copper(II) hexadecafluoro phthalocyanine have been assessed against various bacterial strains. The compound demonstrated selective antibacterial activity with minimum inhibitory concentration (MIC) values comparable to or better than standard antibiotics like doxycycline . Notably, it was effective against both Gram-positive and Gram-negative bacteria.
Photodynamic Therapy (PDT)
This compound has shown promise in PDT applications. Studies indicate that its incorporation into polymeric micelles enhances cellular uptake and photocytotoxicity in tumor models. In vivo experiments demonstrated significant tumor growth inhibition in treated mice .
Comparative Analysis of Biological Activities
Activity | Copper(II) Hexadecafluoro Phthalocyanine | Other Copper(II) Complexes |
---|---|---|
Anticancer Activity | IC50: 5.0 - 7.0 µM | Varies; generally higher IC50 |
Antioxidant Activity | Significant | Minimal |
DNA Interaction | Neutral behavior | Protective by ligands |
Antibacterial Activity | Effective against multiple strains | Varies; often less effective |
Photodynamic Efficacy | High efficacy in tumor models | Variable efficacy |
Case Study 1: Photodynamic Therapy Efficacy
In a controlled study involving CT26 tumor-bearing BALB/c mice treated with polymeric micelles containing copper(II) hexadecafluoro phthalocyanine:
- Results: Complete regression of tumors in 20% of treated subjects.
- Conclusion: The compound’s enhanced photodynamic efficacy is attributed to improved biodistribution and cellular uptake facilitated by micelle encapsulation .
Case Study 2: Antioxidant and Anticancer Synergy
A comparative study evaluated the antioxidant capabilities of copper(II) complexes against their ligands:
Properties
CAS No. |
14916-87-1 |
---|---|
Molecular Formula |
C32CuF16N8 |
Molecular Weight |
863.9 g/mol |
IUPAC Name |
copper;5,6,7,8,14,15,16,17,23,24,25,26,32,33,34,35-hexadecafluoro-2,11,20,37,38,39-hexaza-29,40-diazanidanonacyclo[28.6.1.13,10.112,19.121,28.04,9.013,18.022,27.031,36]tetraconta-1,3,5,7,9,11,13(18),14,16,19(39),20,22(27),23,25,28(38),30(37),31(36),32,34-nonadecaene |
InChI |
InChI=1S/C32F16N8.Cu/c33-9-1-2(10(34)18(42)17(9)41)26-49-25(1)53-27-3-4(12(36)20(44)19(43)11(3)35)29(50-27)55-31-7-8(16(40)24(48)23(47)15(7)39)32(52-31)56-30-6-5(28(51-30)54-26)13(37)21(45)22(46)14(6)38;/q-2;+2 |
InChI Key |
FJAOBQORBYMRNO-UHFFFAOYSA-N |
SMILES |
C12=C(C(=C(C(=C1F)F)F)F)C3=NC4=NC(=NC5=C6C(=C([N-]5)N=C7C8=C(C(=C(C(=C8F)F)F)F)C(=N7)N=C2[N-]3)C(=C(C(=C6F)F)F)F)C9=C4C(=C(C(=C9F)F)F)F.[Cu+2] |
Canonical SMILES |
C12=C(C(=C(C(=C1F)F)F)F)C3=NC4=C5C(=C([N-]4)N=C6C7=C(C(=C(C(=C7F)F)F)F)C(=N6)N=C8C9=C(C(=C(C(=C9F)F)F)F)C(=N8)[N-]C2=N3)C(=C(C(=C5F)F)F)F.[Cu+2] |
Origin of Product |
United States |
Disclaimer and Information on In-Vitro Research Products
Please be aware that all articles and product information presented on BenchChem are intended solely for informational purposes. The products available for purchase on BenchChem are specifically designed for in-vitro studies, which are conducted outside of living organisms. In-vitro studies, derived from the Latin term "in glass," involve experiments performed in controlled laboratory settings using cells or tissues. It is important to note that these products are not categorized as medicines or drugs, and they have not received approval from the FDA for the prevention, treatment, or cure of any medical condition, ailment, or disease. We must emphasize that any form of bodily introduction of these products into humans or animals is strictly prohibited by law. It is essential to adhere to these guidelines to ensure compliance with legal and ethical standards in research and experimentation.