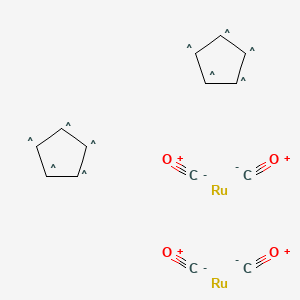
Dicarbonylcyclopentadienylruthenium(II) dimer
- Click on QUICK INQUIRY to receive a quote from our team of experts.
- With the quality product at a COMPETITIVE price, you can focus more on your research.
Overview
Description
Dicarbonylcyclopentadienylruthenium(II) dimer, also known as bis(cyclopentadienylruthenium dicarbonyl) dimer, is a coordination compound with the formula ([Ru(CO)_2(\eta^5-C_5H_5)]_2). This compound is notable for its unique structure, which consists of two ruthenium atoms each bonded to a cyclopentadienyl ring and two carbonyl groups.
Preparation Methods
Synthetic Routes and Reaction Conditions: Dicarbonylcyclopentadienylruthenium(II) dimer can be synthesized through the reaction of ruthenium trichloride with cyclopentadiene in the presence of carbon monoxide. The reaction typically occurs under high-pressure conditions and elevated temperatures to facilitate the formation of the dimeric structure .
Industrial Production Methods: While specific industrial production methods are not extensively documented, the synthesis generally involves the use of high-pressure reactors and specialized equipment to handle the reactive intermediates and ensure the purity of the final product .
Chemical Reactions Analysis
Types of Reactions: Dicarbonylcyclopentadienylruthenium(II) dimer undergoes various types of chemical reactions, including:
Oxidation: The compound can be oxidized to form higher oxidation state species.
Reduction: It can be reduced to form lower oxidation state species.
Substitution: The carbonyl ligands can be substituted with other ligands such as phosphines or amines.
Common Reagents and Conditions:
Oxidation: Common oxidizing agents include oxygen and peroxides.
Reduction: Reducing agents such as hydrogen gas or hydrides are typically used.
Substitution: Ligand substitution reactions often require the presence of a base and are conducted under inert atmosphere conditions.
Major Products: The major products formed from these reactions depend on the specific reagents and conditions used. For example, substitution reactions can yield a variety of ruthenium complexes with different ligands .
Scientific Research Applications
Dicarbonylcyclopentadienylruthenium(II) dimer has a wide range of applications in scientific research, including:
Chemistry: It is used as a catalyst in various organic reactions, including hydrogenation and carbonylation processes.
Biology: The compound has been studied for its potential biological activity and interactions with biomolecules.
Medicine: Research is ongoing into its potential use in medicinal chemistry, particularly in the development of anticancer agents.
Mechanism of Action
The mechanism by which dicarbonylcyclopentadienylruthenium(II) dimer exerts its effects involves the coordination of the ruthenium center with various substrates. The compound can facilitate the activation of small molecules through oxidative addition, reductive elimination, and ligand exchange processes. These reactions are mediated by the unique electronic and steric properties of the cyclopentadienyl and carbonyl ligands .
Comparison with Similar Compounds
Dicarbonylcyclopentadienylrhodium(I) dimer: Similar structure but with rhodium instead of ruthenium.
Dicarbonylcyclopentadienyliridium(I) dimer: Similar structure but with iridium instead of ruthenium.
Dicarbonylcyclopentadienyliron(II) dimer: Similar structure but with iron instead of ruthenium.
Uniqueness: Dicarbonylcyclopentadienylruthenium(II) dimer is unique due to the specific electronic properties of ruthenium, which confer distinct reactivity and stability compared to its rhodium, iridium, and iron analogs. This makes it particularly valuable in catalytic applications where these properties are advantageous .
Biological Activity
Dicarbonylcyclopentadienylruthenium(II) dimer (C₁₄H₁₀O₄Ru₂) is an organometallic compound that has garnered attention for its potential biological activities, particularly in medicinal chemistry and catalysis. This article explores its biological activity, mechanisms of action, and relevant case studies, providing a comprehensive overview based on diverse research findings.
Overview of this compound
This compound is characterized by its unique structure, which includes cyclopentadienyl and carbonyl ligands. It has been studied for various applications in chemistry and biology, including its role as a catalyst in organic reactions and its potential therapeutic effects.
Mechanisms of Biological Activity
The biological activity of this compound is primarily attributed to its ability to coordinate with biomolecules, facilitating various biochemical interactions. Key mechanisms include:
- Oxidative Addition : The compound can activate small molecules through oxidative addition, which is crucial for many biochemical transformations.
- Reductive Elimination : This process allows the release of products from the metal center, influencing the reactivity and selectivity in biological systems.
- Ligand Exchange : The substitution of carbonyl ligands with other biomolecules can enhance the compound's interaction with cellular targets.
Biological Activity Studies
Research has indicated several areas where this compound exhibits biological activity:
- Anticancer Properties : Preliminary studies suggest that this compound may act as an anticancer agent by inducing apoptosis in cancer cells. The mechanism appears to involve the generation of reactive oxygen species (ROS), leading to oxidative stress and subsequent cell death.
- Enzyme Interaction : The compound's ability to interact with enzymes has been investigated, revealing potential inhibitory effects on certain enzymatic pathways that are crucial for tumor growth and proliferation.
- Cellular Uptake : Studies have shown that this compound can be taken up by cells, suggesting potential for targeted drug delivery systems in cancer therapy.
Case Study 1: Anticancer Activity
A study conducted on various cancer cell lines demonstrated that treatment with this compound resulted in significant cytotoxicity. The IC50 values for different cell lines ranged from 5 to 15 µM, indicating a potent effect against these cells. The study highlighted the importance of the compound's metal center in mediating its biological effects.
Case Study 2: Enzyme Inhibition
Research focused on the inhibition of specific enzymes involved in cancer metabolism showed that this compound could inhibit lactate dehydrogenase (LDH), an enzyme critical for anaerobic respiration in tumors. This inhibition was quantified using enzymatic assays, revealing a dose-dependent response with an IC50 value of approximately 10 µM.
Data Table
The following table summarizes key findings from studies on the biological activity of this compound:
Study Focus | Cell Line/Target | IC50 (µM) | Mechanism |
---|---|---|---|
Anticancer Activity | A549 (Lung Cancer) | 7 | Induction of apoptosis via ROS generation |
Anticancer Activity | HeLa (Cervical Cancer) | 10 | Apoptosis induction |
Enzyme Inhibition | Lactate Dehydrogenase | 10 | Competitive inhibition |
Cellular Uptake | Various Cancer Cell Lines | N/A | Confirmed via fluorescence microscopy |
Properties
InChI |
InChI=1S/2C5H5.4CO.2Ru/c2*1-2-4-5-3-1;4*1-2;;/h2*1-5H;;;;;; |
Source
|
---|---|---|
Source | PubChem | |
URL | https://pubchem.ncbi.nlm.nih.gov | |
Description | Data deposited in or computed by PubChem | |
InChI Key |
OYBGZXRXNFYOGP-UHFFFAOYSA-N |
Source
|
Source | PubChem | |
URL | https://pubchem.ncbi.nlm.nih.gov | |
Description | Data deposited in or computed by PubChem | |
Canonical SMILES |
[C-]#[O+].[C-]#[O+].[C-]#[O+].[C-]#[O+].[CH]1[CH][CH][CH][CH]1.[CH]1[CH][CH][CH][CH]1.[Ru].[Ru] |
Source
|
Source | PubChem | |
URL | https://pubchem.ncbi.nlm.nih.gov | |
Description | Data deposited in or computed by PubChem | |
Molecular Formula |
C14H10O4Ru2 |
Source
|
Source | PubChem | |
URL | https://pubchem.ncbi.nlm.nih.gov | |
Description | Data deposited in or computed by PubChem | |
Molecular Weight |
444.4 g/mol |
Source
|
Source | PubChem | |
URL | https://pubchem.ncbi.nlm.nih.gov | |
Description | Data deposited in or computed by PubChem | |
CAS No. |
12132-87-5 |
Source
|
Record name | Di-μ-carbonyl dicarbonyldicyclo pentadienyl diruthenium(II) | |
Source | European Chemicals Agency (ECHA) | |
URL | https://echa.europa.eu/information-on-chemicals | |
Description | The European Chemicals Agency (ECHA) is an agency of the European Union which is the driving force among regulatory authorities in implementing the EU's groundbreaking chemicals legislation for the benefit of human health and the environment as well as for innovation and competitiveness. | |
Explanation | Use of the information, documents and data from the ECHA website is subject to the terms and conditions of this Legal Notice, and subject to other binding limitations provided for under applicable law, the information, documents and data made available on the ECHA website may be reproduced, distributed and/or used, totally or in part, for non-commercial purposes provided that ECHA is acknowledged as the source: "Source: European Chemicals Agency, http://echa.europa.eu/". Such acknowledgement must be included in each copy of the material. ECHA permits and encourages organisations and individuals to create links to the ECHA website under the following cumulative conditions: Links can only be made to webpages that provide a link to the Legal Notice page. | |
Q1: What makes Bis(cyclopentadienylruthenium dicarbonyl) dimer a promising catalyst for hydrophosphination reactions?
A1: Bis(cyclopentadienylruthenium dicarbonyl) dimer, often denoted as [CpRu(CO)2]2, displays several advantageous properties as a catalyst for hydrophosphination reactions []:
- High Efficiency: The research demonstrates that [CpRu(CO)2]2 effectively catalyzes the addition of both primary and secondary phosphines to styrene derivatives and Michael acceptors, even at low catalyst loadings (0.1 mol%) [].
- Mild Reaction Conditions: The photocatalytic nature of the reactions allows them to proceed under mild conditions using a commercially available UV-A 9W lamp, simplifying the experimental setup and potentially reducing unwanted side reactions [].
- Improved Performance over Iron Analogues: Compared to the commonly used [CpFe(CO)2]2 catalyst, [CpRu(CO)2]2 exhibits superior performance, achieving faster reaction rates with lower catalyst loadings [].
Q2: What is the proposed mechanism of action for Bis(cyclopentadienylruthenium dicarbonyl) dimer in these hydrophosphination reactions?
A2: While the research paper doesn't delve into the specific mechanistic details, it suggests that the reactions proceed through a photoactivated pathway []. Based on this and knowledge of similar systems, a plausible mechanism likely involves:
Disclaimer and Information on In-Vitro Research Products
Please be aware that all articles and product information presented on BenchChem are intended solely for informational purposes. The products available for purchase on BenchChem are specifically designed for in-vitro studies, which are conducted outside of living organisms. In-vitro studies, derived from the Latin term "in glass," involve experiments performed in controlled laboratory settings using cells or tissues. It is important to note that these products are not categorized as medicines or drugs, and they have not received approval from the FDA for the prevention, treatment, or cure of any medical condition, ailment, or disease. We must emphasize that any form of bodily introduction of these products into humans or animals is strictly prohibited by law. It is essential to adhere to these guidelines to ensure compliance with legal and ethical standards in research and experimentation.