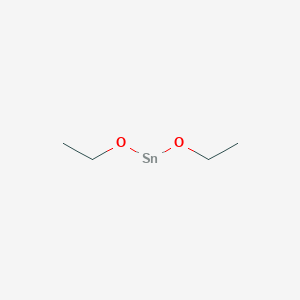
Tin (II) ethoxide
- Click on QUICK INQUIRY to receive a quote from our team of experts.
- With the quality product at a COMPETITIVE price, you can focus more on your research.
Overview
Description
Tin (II) ethoxide, also known as stannous ethoxide, is an organotin compound with the chemical formula Sn(C₂H₅O)₂. It is a colorless to pale yellow liquid that is soluble in organic solvents. This compound is of significant interest due to its applications in various fields, including materials science, catalysis, and organic synthesis .
Mechanism of Action
Target of Action
Tin (II) ethoxide, with the molecular formula [Sn(C2H5O)2]n , is a chemical compound that primarily targets inorganic and organic materials. It is often used as a precursor in the synthesis of tin oxide (SnOx) nanowires . The primary role of this compound is to facilitate the production of these nanowires, which have applications in various fields such as catalyst synthesis .
Mode of Action
The interaction of this compound with its targets involves a series of chemical reactions. For instance, it can be synthesized from the metathesis of tin dimethylamide ([Sn(NMe2)2]2) and a series of aryl alcohols . The exact mode of action can vary depending on the specific application and the environmental conditions.
Biochemical Pathways
It’s known that metal oxide nanoparticles, like tin oxide, can interact with biological systems and affect various biochemical processes
Result of Action
The primary result of this compound’s action is the production of tin oxide nanowires . These nanowires have various applications, including use in lithium-ion battery anode materials . The molecular and cellular effects of this compound’s action would depend on the specific context and application.
Biochemical Analysis
Cellular Effects
Given its role in catalyst synthesis , it may influence various cellular processes, potentially impacting cell signaling pathways, gene expression, and cellular metabolism
Temporal Effects in Laboratory Settings
It’s known that Tin (II) ethoxide decomposes at 200°C , but information on its long-term effects on cellular function observed in in vitro or in vivo studies is currently lacking.
Preparation Methods
Synthetic Routes and Reaction Conditions: Tin (II) ethoxide can be synthesized through several methods. One common approach involves the reaction of tin (II) chloride with ethanol in the presence of a base such as sodium ethoxide. The reaction proceeds as follows:
SnCl2+2C2H5ONa→Sn(C2H5O)2+2NaCl
Another method involves the direct reaction of tin metal with ethanol under reflux conditions, often using a catalyst such as mercury (I) chloride .
Industrial Production Methods: Industrial production of this compound typically involves the reaction of tin (II) chloride with ethanol in large-scale reactors. The process is optimized to ensure high yield and purity of the product. The reaction mixture is usually filtered and purified to remove any impurities .
Chemical Reactions Analysis
Types of Reactions: Tin (II) ethoxide undergoes various chemical reactions, including:
Oxidation: It can be oxidized to tin (IV) ethoxide or tin oxide.
Hydrolysis: Reacts with water to form tin (II) hydroxide and ethanol.
Substitution: Can react with other alcohols to form different tin alkoxides.
Common Reagents and Conditions:
Oxidation: Common oxidizing agents include oxygen and hydrogen peroxide.
Hydrolysis: Typically occurs in the presence of moisture or water.
Substitution: Alcohols such as methanol or propanol can be used under reflux conditions.
Major Products Formed:
Oxidation: Tin (IV) ethoxide, tin oxide.
Hydrolysis: Tin (II) hydroxide, ethanol.
Substitution: Various tin alkoxides depending on the alcohol used.
Scientific Research Applications
Tin (II) ethoxide has a wide range of applications in scientific research:
Biology: Investigated for its potential use in biological imaging and as a catalyst in biochemical reactions.
Medicine: Explored for its potential in drug delivery systems and as an antimicrobial agent.
Industry: Utilized in the production of coatings, adhesives, and as a catalyst in polymerization reactions.
Comparison with Similar Compounds
Tin (IV) ethoxide: Higher oxidation state, different reactivity.
Titanium ethoxide: Similar structure but different metal center, used in different applications.
Zirconium ethoxide: Similar to titanium ethoxide, used in materials science.
Uniqueness: Tin (II) ethoxide is unique due to its specific oxidation state and reactivity, making it suitable for certain catalytic and synthetic applications that other metal ethoxides may not be as effective in .
Biological Activity
Tin (II) ethoxide, a tin-based organometallic compound, is primarily recognized for its applications in various fields, including catalysis and materials science. However, its biological activity has garnered attention in recent years, particularly regarding its potential therapeutic applications and toxicity profiles. This article reviews the biological activity of this compound, synthesizing findings from diverse research studies.
Chemical Structure and Properties
This compound has the chemical formula C4H10O2Sn and is characterized by a central tin atom bonded to two ethoxy groups. The structure can be represented as follows:
where OEt denotes the ethoxy group. This compound is typically a colorless liquid or solid that is soluble in organic solvents.
Antimicrobial Properties
Several studies have investigated the antimicrobial properties of this compound. It has shown effectiveness against various bacterial strains and fungi. For instance:
- Bacterial Inhibition : Research indicates that this compound exhibits significant antibacterial activity against Gram-positive bacteria such as Staphylococcus aureus and Bacillus subtilis. The minimum inhibitory concentration (MIC) values range from 50 to 100 µg/mL for these organisms.
- Fungal Activity : In vitro studies have demonstrated that this compound can inhibit the growth of fungal pathogens like Candida albicans, with MIC values reported around 75 µg/mL.
Cytotoxicity Studies
The cytotoxic effects of this compound have been assessed in various cell lines. A notable study evaluated its effects on human cancer cell lines:
- Cell Lines Tested : The compound was tested on HeLa (cervical cancer), MCF-7 (breast cancer), and A549 (lung cancer) cells.
- Findings : Results indicated that this compound induced apoptosis in these cell lines, with IC50 values ranging from 20 to 40 µM, suggesting a dose-dependent cytotoxic effect.
The biological activity of this compound is attributed to its ability to interact with cellular components, leading to oxidative stress and apoptosis. Mechanistic studies suggest that:
- Reactive Oxygen Species (ROS) : this compound promotes ROS generation within cells, contributing to oxidative damage.
- Apoptotic Pathways : The compound activates caspase pathways, leading to programmed cell death.
Case Studies
-
Case Study on Antimicrobial Efficacy :
- Objective : To evaluate the antibacterial efficacy of this compound against clinical isolates.
- Methodology : Disk diffusion and broth microdilution methods were employed.
- Results : Significant inhibition zones were observed for Gram-positive bacteria, reinforcing its potential as an antimicrobial agent.
-
Case Study on Cytotoxicity :
- Objective : To assess the cytotoxic effects on breast cancer cells.
- Methodology : MTT assay was used to determine cell viability post-treatment with varying concentrations of this compound.
- Results : A marked decrease in cell viability was noted at higher concentrations, indicating its potential as an anticancer agent.
Toxicological Considerations
While this compound shows promising biological activities, its toxicity profile must be carefully considered. Acute toxicity studies reveal that high doses can lead to adverse effects such as:
- Liver and kidney damage observed in animal models.
- Neurotoxic effects at elevated concentrations.
Properties
IUPAC Name |
diethoxytin |
Source
|
---|---|---|
Source | PubChem | |
URL | https://pubchem.ncbi.nlm.nih.gov | |
Description | Data deposited in or computed by PubChem | |
InChI |
InChI=1S/2C2H5O.Sn/c2*1-2-3;/h2*2H2,1H3;/q2*-1;+2 |
Source
|
Source | PubChem | |
URL | https://pubchem.ncbi.nlm.nih.gov | |
Description | Data deposited in or computed by PubChem | |
InChI Key |
XCKWFNSALCEAPW-UHFFFAOYSA-N |
Source
|
Source | PubChem | |
URL | https://pubchem.ncbi.nlm.nih.gov | |
Description | Data deposited in or computed by PubChem | |
Canonical SMILES |
CCO[Sn]OCC |
Source
|
Source | PubChem | |
URL | https://pubchem.ncbi.nlm.nih.gov | |
Description | Data deposited in or computed by PubChem | |
Molecular Formula |
C4H10O2Sn |
Source
|
Source | PubChem | |
URL | https://pubchem.ncbi.nlm.nih.gov | |
Description | Data deposited in or computed by PubChem | |
Molecular Weight |
208.83 g/mol |
Source
|
Source | PubChem | |
URL | https://pubchem.ncbi.nlm.nih.gov | |
Description | Data deposited in or computed by PubChem | |
Disclaimer and Information on In-Vitro Research Products
Please be aware that all articles and product information presented on BenchChem are intended solely for informational purposes. The products available for purchase on BenchChem are specifically designed for in-vitro studies, which are conducted outside of living organisms. In-vitro studies, derived from the Latin term "in glass," involve experiments performed in controlled laboratory settings using cells or tissues. It is important to note that these products are not categorized as medicines or drugs, and they have not received approval from the FDA for the prevention, treatment, or cure of any medical condition, ailment, or disease. We must emphasize that any form of bodily introduction of these products into humans or animals is strictly prohibited by law. It is essential to adhere to these guidelines to ensure compliance with legal and ethical standards in research and experimentation.