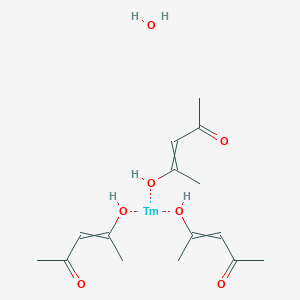
Thulium(III) acetylacetonate
- Click on QUICK INQUIRY to receive a quote from our team of experts.
- With the quality product at a COMPETITIVE price, you can focus more on your research.
Overview
Description
Thulium(III) acetylacetonate is a coordination compound with the chemical formula Tm(C₅H₇O₂)₃. It is a complex formed between thulium, a rare earth element, and acetylacetone, an organic ligand. This compound is known for its unique properties and applications in various fields, including chemistry, biology, and industry .
Preparation Methods
Synthetic Routes and Reaction Conditions: Thulium(III) acetylacetonate can be synthesized by reacting thulium hydroxide with acetylacetone. The reaction typically involves dissolving thulium hydroxide in an acidic solution and then adding acetylacetone. The pH of the solution is gradually raised until the complex precipitates .
Industrial Production Methods: In industrial settings, the preparation of this compound follows similar principles but on a larger scale. The process involves careful control of reaction conditions to ensure high purity and yield. The compound is often produced in a controlled environment to prevent contamination and ensure consistency .
Chemical Reactions Analysis
Solid-State Reactions
Thulium(III) acetylacetonate participates in high-temperature solid-state reactions to form mixed-metal oxides. For example:
Reaction with Iron Acetylacetonate :
At 300°C, Tm(acac)₃ reacts with iron acetylacetonate (Fe(acac)₃) to yield hexagonal TmFeO₃ crystals . This reaction proceeds via thermal decomposition of the precursors, followed by oxide formation.
Reactants | Conditions | Product | Crystal Structure |
---|---|---|---|
Tm(acac)₃ + Fe(acac)₃ | 300°C, solid-state | TmFeO₃ | Hexagonal |
Ligand Substitution Reactions
The acetylacetonate ligands in Tm(acac)₃ can be replaced by stronger coordinating agents. A notable example involves trifluoroacetic acid (TFA) :
Reaction with Trifluoroacetic Acid :
Tm(acac)₃ reacts with TFA to form thulium trifluoroacetate (Tm(CF₃COO)₃), releasing acetylacetone as a byproduct . This substitution is driven by the higher acidity of TFA compared to acetylacetone.
Reactants | Conditions | Products |
---|---|---|
Tm(acac)₃ + CF₃COOH | Room temperature | Tm(CF₃COO)₃ + 3 CH₃COCH₂COCH₃ |
Thermal Decomposition
Thermogravimetric analysis (TGA) of Tm(acac)₃ reveals a multi-step decomposition pathway:
-
Step 1 : Loss of hydrated water molecules below 150°C.
-
Step 2 : Decomposition of acetylacetonate ligands between 200–400°C, forming intermediate oxo-clusters.
-
Step 3 : Final decomposition to Tm₂O₃ (thulium(III) oxide) above 600°C .
Comparative Reactivity with Other Lanthanide Acetylacetonates
The reactivity of Tm(acac)₃ aligns with trends observed in other lanthanide acetylacetonates, though its smaller ionic radius (Tm³⁺: 0.994 Å) influences reaction kinetics and product stability.
Compound | Ionic Radius (Å) | Preferred Reaction Type | Typical Products |
---|---|---|---|
Tm(acac)₃ | 0.994 | Ligand substitution | Oxides, trifluoroacetates |
Yb(acac)₃ | 1.008 | Thermal decomposition | Yb₂O₃ |
Er(acac)₃ | 1.030 | Redox reactions | ErOCl |
Key Research Findings
Scientific Research Applications
Chemical Applications
Catalysis
Thulium(III) acetylacetonate serves as an effective catalyst in organic synthesis, facilitating various reactions due to its ability to form stable complexes. It is particularly noted for its role in:
- Organic Reactions : It promotes reactions like aldol condensation and Michael additions, enhancing reaction rates and selectivity.
- Nanostructure Fabrication : Employed in chemical vapor deposition (CVD) processes for creating carbon nanostructures, this compound aids in the controlled growth of thin films .
Biological Applications
Imaging Agents
The compound is being explored as a contrast agent in magnetic resonance imaging (MRI). Its paramagnetic properties enhance imaging quality by improving signal intensity, making it a valuable tool in medical diagnostics .
Drug Delivery Systems
this compound has potential applications in targeted drug delivery systems. Its ability to form stable complexes allows for the encapsulation of therapeutic agents, enabling more efficient delivery to specific sites within the body .
Anticancer Activity
Preliminary studies indicate that thulium compounds may exhibit cytotoxic effects against cancer cells. Research on triple-negative breast cancer cells has shown that treatment with this compound can increase apoptosis rates, suggesting its potential as an anticancer agent .
Industrial Applications
Thin Film Production
this compound is utilized in the production of thin films through atomic layer deposition (ALD). Its solubility in organic solvents makes it an ideal precursor for creating high-quality films used in electronic and optoelectronic devices .
Case Study 1: Cancer Cell Studies
A study investigated the effects of this compound on triple-negative breast cancer cells. The results indicated that treatment led to increased apoptosis rates compared to controls, highlighting its potential as an anticancer agent .
Case Study 2: Nanoparticle Research
Research demonstrated that incorporating this compound into nanoparticles improved their stability and drug-loading capacity. This enhancement was shown to increase therapeutic efficacy in preclinical models, suggesting promising applications in drug delivery .
Mechanism of Action
The mechanism of action of thulium(III) acetylacetonate involves its ability to form stable coordination complexes. The acetylacetonate ligands bind to the thulium ion, creating a stable chelate ring. This stability allows the compound to participate in various chemical reactions and processes. The molecular targets and pathways involved depend on the specific application, such as catalysis or imaging .
Comparison with Similar Compounds
- Ytterbium(III) acetylacetonate
- Erbium(III) acetylacetonate
- Lutetium(III) acetylacetonate
Comparison: Thulium(III) acetylacetonate is unique due to its specific electronic configuration and the resulting properties. Compared to ytterbium(III) and erbium(III) acetylacetonates, this compound exhibits different reactivity and stability profiles. Its applications in imaging and catalysis are also distinct, making it a valuable compound in various research fields .
Biological Activity
Thulium(III) acetylacetonate (Tm(acac)₃) is a coordination compound formed from thulium, a rare earth element, and acetylacetone, an organic ligand. This compound has garnered interest in various fields, particularly due to its unique biological activities and potential applications in medicinal chemistry and materials science.
This compound has the chemical formula Tm(C₅H₇O₂)₃. The compound exhibits coordination chemistry where the acetylacetonate ligands form stable chelate complexes with the thulium ion. This interaction is significant as it influences the compound's biological activity.
- Target Interactions : Tm(acac)₃ interacts with various biological targets, including enzymes and cellular structures. Its mode of action is primarily through coordination chemistry, forming six-membered chelate rings that stabilize interactions with biological macromolecules.
- Biochemical Pathways : The compound has been implicated in various catalytic reactions that can influence metabolic pathways, although specific pathways remain under investigation.
Biological Applications
This compound has shown potential in several biological applications:
- Imaging Agents : It is being explored as a contrast agent in magnetic resonance imaging (MRI), leveraging its paramagnetic properties to enhance imaging quality .
- Drug Delivery Systems : Research indicates its utility in targeted drug delivery systems, where it can facilitate the transport of therapeutic agents to specific sites within the body.
- Anticancer Activity : Preliminary studies suggest that thulium compounds may exhibit cytotoxic effects against cancer cells, possibly through mechanisms involving oxidative stress and apoptosis induction .
Synthesis and Characterization
This compound can be synthesized by reacting thulium hydroxide with acetylacetone in an organic solvent. The resulting compound has been characterized using various techniques, including X-ray crystallography and spectroscopy, confirming its structural integrity and stability under physiological conditions .
Case Study Analysis
- Cancer Cell Studies : A study investigated the effects of Tm(acac)₃ on triple-negative breast cancer cells. The results indicated that treatment with this compound led to increased apoptosis rates compared to controls, suggesting its potential as an anticancer agent .
- Nanoparticle Research : Tm(acac)₃ has been utilized in nanoparticle synthesis for drug delivery applications. The incorporation of this compound into nanoparticles improved their stability and drug-loading capacity, enhancing therapeutic efficacy in preclinical models.
Comparison with Similar Compounds
Compound | Biological Activity | Applications |
---|---|---|
Ytterbium(III) acetylacetonate | Limited anticancer properties | Imaging |
Erbium(III) acetylacetonate | Moderate cytotoxicity | Drug delivery |
This compound | Promising anticancer activity | MRI contrast agent, drug delivery |
This compound stands out due to its unique properties that enable enhanced imaging capabilities and potential therapeutic applications compared to other lanthanide acetylacetonates.
Q & A
Basic Research Questions
Q. What are the optimal synthesis methods for Thulium(III) acetylacetonate, and how do reaction conditions influence crystal structure and purity?
- Methodology : this compound is typically synthesized via solvothermal methods using TmCl₃·xH₂O and acetylacetone in ethanol or methanol under reflux. Purity is ensured by controlling stoichiometric ratios (e.g., 1:3 metal-to-ligand ratio) and reaction time (12–24 hours). Recrystallization from ethanol or supercritical CO₂ (to avoid solvent residues) is recommended for high-purity crystals .
- Key parameters : Temperature (80–120°C), pH (adjusted with NH₃ or NaOH), and solvent polarity significantly affect ligand coordination and crystallinity. Elemental analysis (C, H, O) and FT-IR (to confirm β-diketonate ligand bonding at ~1520 cm⁻¹ and ~1380 cm⁻¹) are critical for validation .
Q. How can researchers characterize the ligand coordination environment in this compound complexes?
- Methodology : Use a combination of:
- X-ray diffraction (XRD) : To determine crystal structure and confirm octahedral coordination of Tm³⁺ by three bidentate acetylacetonate ligands.
- FT-IR spectroscopy : To identify symmetric/asymmetric C-O stretching (1520–1600 cm⁻¹) and C-H bending modes (1250–1380 cm⁻¹) of the acetylacetonate ligand.
- Elemental analysis : To verify stoichiometry (e.g., Tm:C ratio) and hydration state (e.g., trihydrate vs. anhydrous forms) .
Q. What are the primary challenges in maintaining the stability of this compound during storage and experimentation?
- Methodology :
- Storage : Store in airtight containers under inert gas (Ar/N₂) to prevent hydrolysis or oxidation. Hydrated forms (e.g., trihydrate) are more stable than anhydrous variants but may require desiccant packs.
- Handling : Avoid prolonged exposure to moisture or UV light, which can degrade ligand-metal bonds. Thermogravimetric analysis (TGA) under N₂ can assess thermal stability (decomposition typically occurs above 200°C) .
Advanced Research Questions
Q. How can researchers resolve contradictions in reported ligand coordination numbers for this compound across different studies?
- Methodology : Discrepancies (e.g., 2.5 vs. 3 ligands per Tm³⁺) arise from hydration states or synthesis conditions. To address this:
- Conduct synchrotron XRD to resolve crystal structures at atomic resolution.
- Use extended X-ray absorption fine structure (EXAFS) to quantify ligand-metal distances and coordination geometry.
- Compare elemental analysis data with computational models (e.g., density functional theory) to validate stoichiometry .
Q. What experimental strategies can optimize this compound’s performance as a precursor for doped nanomaterials?
- Methodology : For applications like Tm-doped oxides or nanoparticles:
- Solvothermal decomposition : Heat this compound in a high-boiling solvent (e.g., oleylamine) at 250–300°C to yield Tm₂O₃ nanoparticles.
- Co-doping : Combine with transition metal acetylacetonates (e.g., Co, Cu) to tune optical/magnetic properties. Monitor phase purity via XRD and crystallite size via TEM (target: <50 nm) .
Q. How can researchers design experiments to probe the redox activity of this compound in catalytic systems?
- Methodology :
- Cyclic voltammetry : Measure redox potentials in non-aqueous solvents (e.g., acetonitrile) with a Ag/Ag⁺ reference electrode. Tm³⁺/Tm²⁺ transitions typically occur at -2.1 V to -2.5 V vs. SHE.
- Catalytic testing : Use hydrogenation or oxidation reactions (e.g., CO → CO₂) with GC-MS to track product yields. Compare activity with other lanthanide acetylacetonates (e.g., Ho, Er) to identify ligand field effects .
Q. Data Contradiction and Analysis
Q. Why do studies report conflicting data on the thermal decomposition pathways of this compound?
- Resolution : Variations arise from differences in hydration states or atmospheric conditions (e.g., O₂ vs. N₂). To reconcile:
- Perform simultaneous TGA-DSC under controlled atmospheres to map decomposition steps (e.g., ligand loss at 200–250°C, oxide formation >400°C).
- Cross-reference with mass spectrometry (MS) to identify gaseous byproducts (e.g., CO₂, H₂O) .
Q. Methodological Best Practices
- Experimental design : Use triplicate samples and include blank runs (e.g., ligand-only controls) to isolate metal-specific effects.
- Data reporting : Adhere to standards in materials science (e.g., reporting crystallite size via Scherrer equation in XRD) and validate with multiple techniques (e.g., TEM for nanoparticle size) .
Properties
CAS No. |
14589-44-7 |
---|---|
Molecular Formula |
C15H21O6Tm |
Molecular Weight |
466.26 g/mol |
IUPAC Name |
pentane-2,4-dione;thulium(3+) |
InChI |
InChI=1S/3C5H7O2.Tm/c3*1-4(6)3-5(2)7;/h3*3H,1-2H3;/q3*-1;+3 |
InChI Key |
QTKHRYPCYVXQDE-UHFFFAOYSA-N |
SMILES |
CC(=CC(=O)C)O.CC(=CC(=O)C)O.CC(=CC(=O)C)O.O.[Tm] |
Canonical SMILES |
CC(=O)[CH-]C(=O)C.CC(=O)[CH-]C(=O)C.CC(=O)[CH-]C(=O)C.[Tm+3] |
Origin of Product |
United States |
Disclaimer and Information on In-Vitro Research Products
Please be aware that all articles and product information presented on BenchChem are intended solely for informational purposes. The products available for purchase on BenchChem are specifically designed for in-vitro studies, which are conducted outside of living organisms. In-vitro studies, derived from the Latin term "in glass," involve experiments performed in controlled laboratory settings using cells or tissues. It is important to note that these products are not categorized as medicines or drugs, and they have not received approval from the FDA for the prevention, treatment, or cure of any medical condition, ailment, or disease. We must emphasize that any form of bodily introduction of these products into humans or animals is strictly prohibited by law. It is essential to adhere to these guidelines to ensure compliance with legal and ethical standards in research and experimentation.