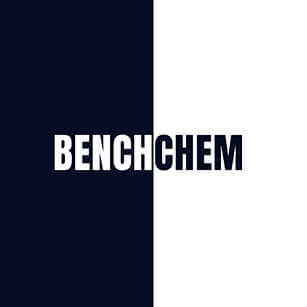
NICKEL PHOSPHATE
- Click on QUICK INQUIRY to receive a quote from our team of experts.
- With the quality product at a COMPETITIVE price, you can focus more on your research.
Overview
Description
Nickel phosphate, with the chemical formula Ni₃(PO₄)₂, is an inorganic compound that appears as a mint green paramagnetic solid. It is insoluble in water and is known for its unique structural properties. The compound can also exist in a hydrated form, Ni₃(PO₄)₂·8H₂O, which is a light green solid. This compound is used in various industrial and scientific applications due to its stability and reactivity .
Mechanism of Action
Target of Action
Nickel phosphate, with its unique electronic structure and chemical stability, primarily targets electrocatalytic reactions . It is synthesized in various compositions, making it highly attractive for a wide range of applications . The primary targets of this compound are the reactions that evolve hydrogen and oxygen, commonly known as water splitting .
Mode of Action
This compound interacts with its targets through a series of complex reactions. Advanced analytic tools combined with theoretical calculations reveal that upon heating, nickel ions are dissolved and coordinated by triphenylphosphine (PPh3), enabling the synthesis of fine-tuned particles, with nickel phosphide phases ranging from Ni3P to Ni2P . This interaction results in changes in the phase composition of the nickel phosphide, which can range from Ni3P to Ni2P .
Biochemical Pathways
This compound affects various biochemical pathways. For instance, it has been found to alter the expression of genes involved in carbon/nitrogen metabolism pathways . Additionally, nickel resistance mechanisms in yeasts and other fungi may involve inactivation of nickel toxicity by the production of extracellular nickel-chelating substances such as glutathione .
Pharmacokinetics
It’s worth noting that nickel nanoparticles have been investigated for their superior ferromagnetic properties, high coercive forces, and chemical stability . These properties could potentially impact the bioavailability of this compound, but more research is needed in this area.
Result of Action
The result of this compound’s action is primarily observed in its catalytic activity. For instance, nickel phosphide phases ranging from Ni3P to Ni2P have shown clear composition-activity trends as electrocatalysts for the hydrogen evolution reaction in acidic media and as anode materials in Li-ion batteries . Moreover, this compound has been found to exhibit high deoxygenation activity, facilitating the integrated catalytic upgradation and pyrolysis of palm kernel shells .
Action Environment
The action of this compound can be influenced by various environmental factors. For instance, the doping of 5% Cu or Mn in either of the nickel phosphide phases yielded a mixture of phases (Ni2P/Ni5P4). Increasing the cu or mn content to 10% resulted in the complete transformation of phase, ie, from Ni2P to pure Ni5P4 and vice versa . This suggests that the presence of certain elements in the environment can significantly influence the action, efficacy, and stability of this compound.
Biochemical Analysis
Biochemical Properties
Nickel Phosphate may interact with various enzymes, proteins, and other biomolecules. For instance, nickel ions are used as enzyme cofactors in organisms from all kingdoms of life, catalyzing a variety of remarkable chemical reactions
Cellular Effects
Nickel-induced toxicity responses have been observed in human epithelial cells . These responses involve changes in the expression and phosphorylation status of critical biochemical pathway regulators .
Molecular Mechanism
It is known that nickel ions can disrupt protein responses and protein response-based biochemical pathways
Metabolic Pathways
Nickel ions are known to be essential cofactors for a variety of enzymes
Preparation Methods
Synthetic Routes and Reaction Conditions: Nickel phosphate can be synthesized through several methods, including hydrothermal synthesis, chemical precipitation, and solid-state reactions. One common method involves the reaction of nickel nitrate with sodium phosphate under controlled conditions to yield this compound and sodium nitrate as a byproduct .
Industrial Production Methods: In industrial settings, this compound is often produced through hydrothermal synthesis, which involves reacting nickel salts with phosphate sources at high temperatures and pressures. This method ensures the formation of high-purity this compound with controlled particle sizes .
Chemical Reactions Analysis
Types of Reactions: Nickel phosphate undergoes various chemical reactions, including:
Oxidation: this compound can be oxidized to form higher oxidation state compounds.
Reduction: It can be reduced to form nickel metal and other lower oxidation state compounds.
Substitution: this compound can participate in substitution reactions where phosphate groups are replaced by other anions.
Common Reagents and Conditions:
Oxidation: Typically involves oxidizing agents such as hydrogen peroxide or potassium permanganate.
Reduction: Reducing agents like hydrogen gas or sodium borohydride are commonly used.
Substitution: Reactions often involve other metal salts or complexing agents under controlled pH and temperature conditions.
Major Products: The major products formed from these reactions include various nickel oxides, reduced nickel species, and substituted phosphate compounds .
Scientific Research Applications
Nickel phosphate has a wide range of applications in scientific research:
Chemistry: Used as a catalyst in various chemical reactions, including hydrogen evolution and oxygen evolution reactions.
Biology: Investigated for its potential use in biological systems due to its biocompatibility and stability.
Medicine: Explored for drug delivery systems and as a component in medical implants.
Industry: Utilized in the production of batteries, supercapacitors, and as a corrosion-resistant coating
Comparison with Similar Compounds
- Cobalt Phosphate
- Iron Phosphate
- Manganese Phosphate
- Zinc Phosphate
Nickel phosphate’s distinct properties and wide range of applications make it a valuable compound in both scientific research and industrial applications.
Properties
CAS No. |
14396-43-1 |
---|---|
Molecular Formula |
H14Ni3O15P2 |
Molecular Weight |
492.13 |
Origin of Product |
United States |
Q1: What are the different morphologies of nickel phosphate reported in the literature?
A1: Researchers have successfully synthesized this compound in various morphologies, including microflower structures [], hierarchically mesoporous rods [], nanotubes [, ], nanoparticles [], lamination structures with ultrathin nanosheets [], and even 3D nano-ice creams []. The morphology significantly influences its properties and applications.
Q2: How does the synthesis method influence the structure of this compound?
A2: Different synthesis techniques, such as hydrothermal methods [, , , ], precipitation methods [, ], and sonochemical methods [, ], impact the final structure and properties of this compound. For example, microwave-assisted hydrothermal synthesis allows for faster reaction rates and can significantly reduce synthesis time compared to conventional hydrothermal methods [].
Q3: Is this compound stable under various conditions?
A3: The stability of this compound depends on its specific form and the surrounding environment. Studies have investigated its performance under acidic and basic conditions [, , ], as well as its thermal stability [, , ]. Doping with other elements like iron [] or cerium [] has been explored to enhance its stability and resistance to acid and base degradation.
Q4: What are the prominent catalytic applications of this compound?
A4: this compound exhibits promising catalytic activity in various reactions. It has shown potential as a catalyst for:
- Electrochemical water oxidation: Iron-doped this compound on nickel foam demonstrates high activity for oxygen evolution reaction (OER) in alkaline solutions []. Doped lithium nickel phosphates have also been explored as catalysts for WOR [].
- Methanol oxidation: Nickel phosphide/graphene nanomaterials, derived from this compound precursors, demonstrate high activity for methanol oxidation [].
- Hydrogen evolution: Platinum-decorated nickel pyrophosphate, synthesized from this compound, displays excellent electrocatalytic performance for the hydrogen evolution reaction (HER) in acidic solutions [].
- Other reactions: this compound nanotubes exhibit catalytic activity in the isomerization of epoxy propane [] and mesoporous this compound shows promise for glycerol conversion to acrolein [].
Q5: How does the structure of this compound affect its catalytic properties?
A5: The structure of this compound significantly influences its catalytic behavior. For instance, nanoporous this compound, such as VSB-5, exhibits shape selectivity and high catalytic activity due to the presence of catalytically active nickel centers dispersed within its porous channels []. Additionally, the presence of specific facets, defects, and surface area can also impact its catalytic performance.
Q6: Have computational methods been employed to study this compound?
A6: Yes, computational studies have provided insights into the electronic properties of different polymorphs of sodium this compound (NaNiPO4) like triphylite and maricite []. Density functional theory (DFT) calculations can predict the electrochemical behavior and stability of these materials, aiding in the design of improved energy storage devices.
Q7: How do modifications to the this compound structure affect its activity?
A7: Structural modifications significantly impact the properties and performance of this compound. For instance:
- Doping: Incorporating elements like iron into the this compound lattice can enhance its catalytic activity towards the oxygen evolution reaction []. Similarly, doping lithium this compound with calcium, magnesium, or yttrium can influence its catalytic behavior in water oxidation [].
- Morphology control: this compound nanotubes show different catalytic activities and selectivities compared to their bulk counterparts in the isomerization of epoxy propane [].
- Surface modifications: Treating nanoporous VSB-5 this compound with NaOH generates active Ni(OH)2 species within its pores, significantly enhancing its catalytic activity for alcohol oxidation [].
Q8: What are some promising applications of this compound in energy storage?
A8: this compound materials show significant potential for application in supercapacitors [, , , ]. Their high specific capacitance, good rate capability, and long cycle life make them attractive electrode materials for high-performance energy storage devices.
Disclaimer and Information on In-Vitro Research Products
Please be aware that all articles and product information presented on BenchChem are intended solely for informational purposes. The products available for purchase on BenchChem are specifically designed for in-vitro studies, which are conducted outside of living organisms. In-vitro studies, derived from the Latin term "in glass," involve experiments performed in controlled laboratory settings using cells or tissues. It is important to note that these products are not categorized as medicines or drugs, and they have not received approval from the FDA for the prevention, treatment, or cure of any medical condition, ailment, or disease. We must emphasize that any form of bodily introduction of these products into humans or animals is strictly prohibited by law. It is essential to adhere to these guidelines to ensure compliance with legal and ethical standards in research and experimentation.