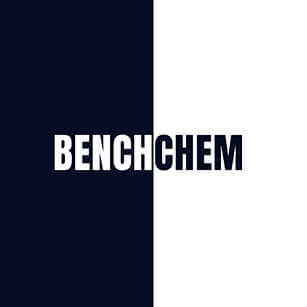
BARIUM FERROCYANIDE
- Click on QUICK INQUIRY to receive a quote from our team of experts.
- With the quality product at a COMPETITIVE price, you can focus more on your research.
Overview
Description
Barium ferrocyanide is an inorganic compound with the molecular formula
C6Ba2FeN6
. It is a coordination complex that contains barium and ferrocyanide ions. This compound is known for its unique chemical properties and applications in various fields, including chemistry, biology, and industry.Mechanism of Action
Target of Action
Barium Ferrocyanide is a complex inorganic compound with the formula Ba2[Fe(CN)6]. It is a mixed-valence compound, where iron exists in two different oxidation states . The primary targets of this compound are the redox-active sites in biochemical systems, where it can participate in electron transfer processes .
Mode of Action
This compound interacts with its targets through redox reactions. In thermoelectrochemical cells, for example, it undergoes redox processes at the electrodes, generating a potential difference and a current . The ratio of the oxidized and reduced states of this compound can significantly affect the power produced .
Biochemical Pathways
This compound affects the redox pathways in biochemical systems. It participates in electron transfer processes, influencing the overall redox balance. The compound’s role in these pathways is primarily associated with its redox properties and its ability to interact with other redox-active species .
Pharmacokinetics
It’s important to note that the bioavailability and pharmacokinetics of any compound depend on various factors, including its chemical properties, the route of administration, and the physiological characteristics of the individual .
Result of Action
The molecular and cellular effects of this compound’s action are primarily related to its redox activity. By participating in electron transfer processes, it can influence the redox state of cells and potentially affect various cellular functions .
Action Environment
Environmental factors can influence the action, efficacy, and stability of this compound. For instance, the formation rates and yields of ferrocyanide, a related compound, are optimal at slightly alkaline conditions (pH 8−9) and moderate temperatures (≈20−30 °C) . These factors could similarly influence the behavior of this compound.
Preparation Methods
Synthetic Routes and Reaction Conditions
Barium ferrocyanide can be synthesized through the reaction of barium chloride with potassium ferrocyanide in an aqueous solution. The reaction typically occurs under controlled conditions to ensure the formation of the desired product. The general reaction is as follows:
BaCl2+K4[Fe(CN)6]→Ba2[Fe(CN)6]+4KCl
Industrial Production Methods
In industrial settings, the production of this compound involves the use of large-scale reactors where barium chloride and potassium ferrocyanide are mixed in precise stoichiometric ratios. The reaction mixture is then filtered to remove any impurities, and the resulting this compound is dried and purified for further use.
Chemical Reactions Analysis
Types of Reactions
Barium ferrocyanide undergoes various chemical reactions, including:
Oxidation: this compound can be oxidized to form ferricyanide compounds.
Reduction: It can be reduced back to ferrocyanide under specific conditions.
Substitution: The cyanide ligands in this compound can be substituted with other ligands in the presence of suitable reagents.
Common Reagents and Conditions
Oxidation: Common oxidizing agents include ferric chloride and hydrogen peroxide.
Reduction: Reducing agents such as sodium borohydride or hydrazine can be used.
Substitution: Ligand substitution reactions often require the presence of transition metal complexes and appropriate solvents.
Major Products Formed
Oxidation: Ferricyanide compounds.
Reduction: Ferrocyanide compounds.
Substitution: Various substituted ferrocyanide complexes.
Scientific Research Applications
Barium ferrocyanide has several scientific research applications, including:
Chemistry: Used as a precursor for the synthesis of other coordination compounds and as a reagent in analytical chemistry.
Biology: Employed in studies involving electron transport and redox reactions in biological systems.
Medicine: Investigated for its potential use in drug delivery systems and as a contrast agent in medical imaging.
Industry: Utilized in the production of pigments, anticaking agents, and as a catalyst in various chemical processes.
Comparison with Similar Compounds
Similar Compounds
Potassium Ferrocyanide: K4[Fe(CN)6]
Sodium Ferrocyanide: Na4[Fe(CN)6]
Calcium Ferrocyanide: Ca2[Fe(CN)6]
Uniqueness
Barium ferrocyanide is unique due to its specific coordination with barium ions, which imparts distinct chemical properties and reactivity compared to other ferrocyanide compounds. Its applications in various fields, such as catalysis and medical imaging, highlight its versatility and importance in scientific research.
Properties
CAS No. |
13821-06-2 |
---|---|
Molecular Formula |
C6Ba2FeN6 |
Molecular Weight |
486.60 g/mol |
IUPAC Name |
barium(2+);iron(2+);hexacyanide |
InChI |
InChI=1S/6CN.2Ba.Fe/c6*1-2;;;/q6*-1;3*+2 |
InChI Key |
NUZIUQUIUFXLAH-UHFFFAOYSA-N |
Canonical SMILES |
[C-]#N.[C-]#N.[C-]#N.[C-]#N.[C-]#N.[C-]#N.[Fe+2].[Ba+2].[Ba+2] |
physical_description |
Cream colored powder; [Alfa Aesar MSDS] |
Origin of Product |
United States |
Q1: What are the advantages of using Barium Ferrocyanide over Lead Ferrocyanide in electrical squibs?
A1: The research indicates that this compound offers several advantages over Lead Ferrocyanide in electrical squib applications:
- Enhanced Safety: BFCN-based squibs demonstrate a higher no-fire current (NFC) compared to LFCN-based squibs []. This higher threshold makes them safer to handle as accidental ignition is less likely.
- Comparable Performance: BFCN demonstrates comparable electrothermal response (ETR) to LFCN, indicating its effectiveness in generating heat upon electrical stimulation [].
- Improved Shelf Life: Accelerated aging tests suggest that BFCN-based squibs possess a reasonable shelf life, ensuring reliable long-term performance [].
Q2: How can the electrothermal response (ETR) technique be used for quality control of this compound-based squibs?
A2: The research highlights the ETR technique as a valuable tool for quality control of BFCN-based squibs []. By analyzing the ETR, manufacturers can:
Disclaimer and Information on In-Vitro Research Products
Please be aware that all articles and product information presented on BenchChem are intended solely for informational purposes. The products available for purchase on BenchChem are specifically designed for in-vitro studies, which are conducted outside of living organisms. In-vitro studies, derived from the Latin term "in glass," involve experiments performed in controlled laboratory settings using cells or tissues. It is important to note that these products are not categorized as medicines or drugs, and they have not received approval from the FDA for the prevention, treatment, or cure of any medical condition, ailment, or disease. We must emphasize that any form of bodily introduction of these products into humans or animals is strictly prohibited by law. It is essential to adhere to these guidelines to ensure compliance with legal and ethical standards in research and experimentation.