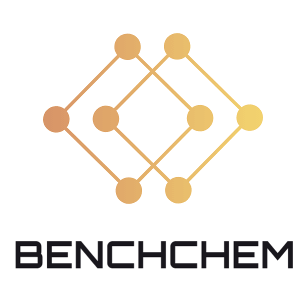
GADOLINIUM SULFIDE
- Click on QUICK INQUIRY to receive a quote from our team of experts.
- With the quality product at a COMPETITIVE price, you can focus more on your research.
Description
Gadolinium Sulfide is a compound of the rare earth metal gadolinium . It is also known as gadolinium sulfoxylate, GOS or Gadox . It is an inorganic compound, a mixed oxide-sulfide of gadolinium . The main use of gadolinium oxysulfide is in ceramic scintillators .
Synthesis Analysis
Europium activated this compound nanoparticles were synthesized by a chemical route by varying the Europium concentration . Two powder preparation routes have been successful for synthesizing Gd2O2S: Pr, Ce, F powder complexes for the ceramic scintillators. These preparations routes are called the halide flux method and the sulfite precipitation method .Molecular Structure Analysis
The molecular structure of this compound was analyzed using X-ray diffraction and scanning electron microscopy . The oxidation state of the Europium ions was elucidated from X-ray absorption near edge structure (XANES) spectra .Chemical Reactions Analysis
Gadolinium exhibits a sluggish reaction when exposed to cold water, while it displays a rapid reaction when in contact with hot water . This reaction leads to the formation of gadolinium hydroxide (Gd(OH)3) and the liberation of hydrogen gas (H2) .Physical and Chemical Properties Analysis
This compound is a white odorless powder . It has a density of 7.32 g/cm3 . It is insoluble in water . This compound exhibits final densities of 99.7% to 99.99% of the theoretical density (7.32 g/cm3) and an average grain size ranging from 5 micrometers to 50 micrometers in dependence with the fabrication procedure .Mechanism of Action
Future Directions
In the last decade, the publications presenting novel physical and chemical aspects of gadolinium-based oxide (Gd2O3) and oxysulfide (Gd2O2S) particles in the micro- or nano-scale have increased, mainly stimulated by the exciting applications of these materials in the biomedical field . The optical properties of Gd-based materials can be enhanced by doping the matrix with other lanthanide ions .
Properties
CAS No. |
12134-74-6 |
---|---|
Molecular Formula |
GdS |
Molecular Weight |
189.32 |
Origin of Product |
United States |
Disclaimer and Information on In-Vitro Research Products
Please be aware that all articles and product information presented on BenchChem are intended solely for informational purposes. The products available for purchase on BenchChem are specifically designed for in-vitro studies, which are conducted outside of living organisms. In-vitro studies, derived from the Latin term "in glass," involve experiments performed in controlled laboratory settings using cells or tissues. It is important to note that these products are not categorized as medicines or drugs, and they have not received approval from the FDA for the prevention, treatment, or cure of any medical condition, ailment, or disease. We must emphasize that any form of bodily introduction of these products into humans or animals is strictly prohibited by law. It is essential to adhere to these guidelines to ensure compliance with legal and ethical standards in research and experimentation.