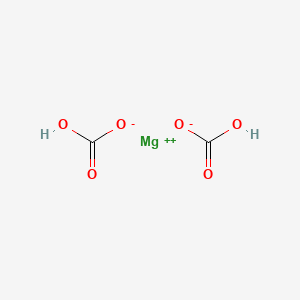
Magnesium bicarbonate
Overview
Description
Magnesium bicarbonate (Mg(HCO₃)₂) is an unstable ionic compound that exists only in aqueous solutions, as it readily decomposes into magnesium carbonate (MgCO₃), carbon dioxide (CO₂), and water (H₂O) in its solid form . It is formed by reacting magnesium hydroxide (Mg(OH)₂) or magnesium carbonate with carbonated water under controlled conditions . Key properties include:
- Solubility: Highly soluble in water, facilitating rapid absorption in biological systems .
- pH: Aqueous solutions are slightly alkaline (pH 8–9), contributing to buffering capacity .
- Biological Role: Essential for mitochondrial function, ATP synthesis, and electrolyte balance .
This compound is primarily used in dietary supplements for its high bioavailability and in water treatment for ion exchange .
Preparation Methods
Laboratory-Scale Synthesis of Magnesium Bicarbonate
Reaction of Magnesium Hydroxide with Carbonic Acid
The most widely documented laboratory method involves reacting magnesium hydroxide (Mg(OH)₂) with carbonic acid (H₂CO₃). Carbonic acid is typically generated in situ by dissolving carbon dioxide (CO₂) in water. The reaction proceeds as follows:
2 + 2\ \text{CO}2 \rightarrow \text{Mg(HCO}3\text{)}2 + \text{H}_2\text{O}
This method requires pressurized CO₂ to enhance solubility and reaction efficiency. A suspension of Mg(OH)₂ is treated with CO₂ at pressures slightly above atmospheric (1.1–1.5 atm) and temperatures between 20°C and 25°C . The resulting solution contains approximately 1.2–1.5% this compound by weight, beyond which precipitation of magnesium carbonate (MgCO₃) occurs .
Key Parameters:
-
CO₂ Pressure : Higher pressures (up to 2 atm) increase reaction rate but necessitate specialized equipment.
-
Temperature : Elevated temperatures (>30°C) accelerate CO₂ dissolution but risk premature decomposition of Mg(HCO₃)₂ into MgCO₃.
-
Purity Control : Residual Mg(OH)₂ is removed via filtration, yielding solutions with >90% Mg(HCO₃)₂ purity .
Double Displacement Using Magnesium Acetate and Sodium Bicarbonate
An alternative laboratory method employs a double displacement reaction between magnesium acetate (Mg(CH₃COO)₂) and sodium bicarbonate (NaHCO₃):
3\text{COO)}2 + 2\ \text{NaHCO}3 \rightarrow \text{Mg(HCO}3\text{)}2 + 2\ \text{CH}3\text{COONa}
This reaction occurs in aqueous media at ambient temperature (20–25°C). Sodium acetate (CH₃COONa) precipitates out, leaving Mg(HCO₃)₂ in solution. The method is favored for its simplicity but requires stoichiometric precision to avoid sodium contamination .
Yield Optimization:
-
Molar Ratio : A 1:2 molar ratio of Mg(CH₃COO)₂ to NaHCO₃ maximizes yield (∼85–90%).
-
Filtration : Centrifugation at 3,000–5,000 rpm removes sodium acetate impurities .
Industrial-Scale Production Methods
Continuous Flow Reactor Systems
Industrial processes prioritize scalability and cost-efficiency. A patented continuous flow system (Figure 1) involves:
-
Carbonic Acid Generation : CO₂ is dissolved in water under pressure (2–3 atm) to form H₂CO₃.
-
Reaction with Mg(OH)₂ Slurry : The acid is mixed with a Mg(OH)₂ slurry (30–70% solids by weight) in a reactor vessel.
-
Rapid Filtration : Calcium carbonate (CaCO₃) impurities, common in industrial-grade Mg(OH)₂, are removed within 10–90 seconds to prevent competitive reactions .
Reaction Dynamics :
2 + 2\ \text{H}2\text{CO}3 \rightarrow \text{Mg(HCO}3\text{)}2 + 2\ \text{H}2\text{O}
Industrial Parameters:
Parameter | Range | Effect on Purity |
---|---|---|
Filtration Time | 10–90 seconds | Reduces CaCO₃ content to <5% |
Temperature | 15–25°C | Prevents MgCO₃ precipitation |
CO₂ Concentration | 1.5–2.5% (w/w) | Optimizes H₂CO₃ availability |
Post-filtration, the Mg(HCO₃)₂ solution is stabilized at pH 6.8–7.2 and concentrated via vacuum evaporation .
Comparative Analysis of Preparation Methods
Yield and Purity Across Methods
Method | Yield (%) | Purity (%) | Scalability | Cost (USD/kg) |
---|---|---|---|---|
Mg(OH)₂ + CO₂ | 85–92 | 90–95 | High | 12–18 |
Mg Acetate + NaHCO₃ | 80–85 | 88–92 | Low | 25–35 |
Industrial Continuous | 90–95 | 95–98 | Very High | 8–14 |
Key Observations :
-
Industrial continuous systems achieve the highest purity (95–98%) due to rapid impurity removal .
-
Laboratory methods using Mg(OH)₂ and CO₂ balance cost and yield but require pressurized equipment .
Challenges and Mitigation Strategies
-
Instability of Mg(HCO₃)₂ : Solutions decompose into MgCO₃, CO₂, and H₂O upon drying or heating >30°C. Stabilizers like EDTA (0.1–0.3%) extend shelf life to 7–10 days.
-
Calcium Contamination : Industrial-grade Mg(OH)₂ often contains CaCO₃. Differential reaction rates (Mg(OH)₂ reacts 10–20× faster than CaCO₃ with H₂CO₃) allow selective filtration .
Recent Advancements in Preparation Technologies
Membrane-Based Purification
Novel nanofiltration membranes (MWCO 200–300 Da) selectively separate Mg(HCO₃)₂ from Ca²⁺ and Na⁺ ions, achieving >99% purity. Pilot-scale trials report 30% reduction in production costs compared to traditional filtration .
Electrochemical Carbonic Acid Generation
Electrolytic cells generate H₂CO₃ directly from CO₂ and water, eliminating the need for pressurized CO₂ tanks. Early-stage systems achieve 85–90% reaction efficiency at 1.5 V and 25°C .
Chemical Reactions Analysis
Magnesium bicarbonate undergoes several types of chemical reactions:
-
Decomposition: : When dried, this compound decomposes into magnesium carbonate, carbon dioxide, and water . [ \text{Mg(HCO}_3\text{)}_2 \rightarrow \text{MgCO}_3 + \text{CO}_2 + \text{H}_2\text{O} ]
-
Reaction with Acids: : this compound reacts with acids to form magnesium salts, carbon dioxide, and water . [ \text{Mg(HCO}_3\text{)}_2 + 2\text{HCl} \rightarrow \text{MgCl}_2 + 2\text{CO}_2 + 2\text{H}_2\text{O} ]
-
Reaction with Bases: : It can also react with bases to form magnesium hydroxide and carbonates . [ \text{Mg(HCO}_3\text{)}_2 + 2\text{NaOH} \rightarrow \text{Mg(OH)}_2 + 2\text{NaHCO}_3 ]
Scientific Research Applications
Health Applications
1. Cardiovascular Health
Magnesium bicarbonate has been studied for its potential benefits in cardiovascular health. A double-blind, placebo-controlled study involving postmenopausal women demonstrated that regular consumption of this compound supplemented spring water led to significant increases in serum magnesium concentrations and urinary pH levels. However, there were no significant changes in biomarkers of bone mineral metabolism or serum lipids over the study period .
2. Bone Health
Research indicates that magnesium plays a crucial role in bone metabolism. Although the aforementioned study did not show significant effects on bone biomarkers, the importance of magnesium in preventing conditions like osteoporosis has been highlighted in other studies. This compound's alkalizing effect may contribute to improved bone density and overall mineral balance .
3. Gastrointestinal Health
This compound is also utilized for its buffering capacity in gastrointestinal applications. It can help neutralize stomach acid, providing relief from conditions such as acid reflux and indigestion. In animal studies, magnesium hydroxide (a related compound) exhibited better buffering capacity than sodium bicarbonate, suggesting potential advantages for magnesium-based formulations .
Environmental Applications
1. Water Treatment
This compound is used in water treatment processes to adjust pH levels and improve the quality of drinking water. It can enhance the mineral content of water, which has been linked to various health benefits, including reduced cardiovascular disease risk .
2. Soil Amendment
The compound is also explored as a soil amendment to improve soil health and nutrient availability. Its application can increase soil pH and provide essential magnesium to plants, promoting better growth and yield.
Industrial Applications
1. Metal Extraction and Purification
This compound finds applications in the extractive metallurgy industry as an acidity balancing agent during metal extraction processes. It helps stabilize pH levels when using acidic organic extractants, improving the efficiency of metal recovery from ores . The process involves mixing this compound with metal solutions to facilitate better extraction rates while minimizing environmental impact.
2. Carbon Capture
Recent innovations have proposed using this compound solutions for carbon capture technologies. The compound can react with carbon dioxide to form stable carbonates, potentially aiding in reducing greenhouse gas emissions from industrial processes .
Case Studies
Mechanism of Action
Magnesium bicarbonate exerts its effects through various mechanisms:
Comparison with Similar Compounds
Sodium Bicarbonate (NaHCO₃)
Key Difference : Sodium bicarbonate is more stable and widely used in industrial and culinary applications, while this compound’s instability limits it to specialized roles in supplements and solutions .
Ammonium Bicarbonate (NH₄HCO₃)
Key Difference : Ammonium bicarbonate’s volatility necessitates chemical stabilizers, whereas this compound’s instability is inherent to its chemical structure .
Magnesium Carbonate (MgCO₃)
Key Difference : Magnesium carbonate’s low solubility and stability make it suitable for solid formulations, contrasting with this compound’s role in liquid-based applications .
Magnesium Hydroxide (Mg(OH)₂)
Key Difference : Magnesium hydroxide’s stability and slow reactivity contrast with this compound’s rapid dissolution and metabolic efficiency .
Calcium Bicarbonate (Ca(HCO₃)₂)
Property | This compound | Calcium Bicarbonate |
---|---|---|
Stability | Unstable in solid form | Even less stable, rarely isolated |
Natural Occurrence | Found in mineral waters | Forms temporary hardness in water |
Key Difference : Both compounds are unstable, but calcium bicarbonate’s transient nature limits its applications compared to this compound’s role in supplements .
Biological Activity
Magnesium bicarbonate is a compound that plays a crucial role in various biological processes within the human body. Its significance stems from its ability to provide magnesium ions, which are essential for numerous physiological functions, including enzyme activation, muscle contraction, and nerve function. This article explores the biological activity of this compound, highlighting its mechanisms of action, health benefits, and relevant research findings.
1. Enzyme Activation and Metabolism
Magnesium is a cofactor for over 300 enzymes involved in critical biochemical reactions. This compound contributes to the activation of enzymes that regulate protein, lipid, and carbohydrate metabolism, thereby facilitating energy production and nutrient utilization in the body .
2. Muscle and Nerve Function
Magnesium is vital for maintaining normal muscle and nerve function. It helps regulate electrical impulses across cell membranes, which is essential for muscle contraction and relaxation as well as neurotransmission . this compound aids in maintaining the electrical charge of cells, particularly in muscles and nerves.
3. Bone Health
Magnesium plays a significant role in bone metabolism by influencing calcium homeostasis. It is involved in the synthesis of bone matrix proteins and helps to prevent osteoporosis by regulating parathyroid hormone levels . Studies indicate that this compound supplementation may enhance bone health by providing a bioavailable source of magnesium .
Health Benefits
1. Cardiovascular Health
this compound has been associated with cardiovascular health benefits. It aids in regulating blood pressure and supports heart muscle function, potentially reducing the risk of cardiovascular diseases . A study indicated that magnesium supplementation could improve lipid profiles and reduce blood pressure in postmenopausal women .
2. Kidney Stone Prevention
Research suggests that this compound can help prevent kidney stones by increasing the solubility of calcium in urine, thus reducing calcium oxalate crystallization . This property makes it beneficial for individuals prone to kidney stones.
3. Lung Function
Emerging studies have suggested that this compound may positively affect lung function. Its role in muscle relaxation can support respiratory function, although further research is needed to establish definitive links .
Case Studies
A double-blind, placebo-controlled study involving 67 postmenopausal women examined the effects of this compound supplemented water over 84 days. The study found significant increases in serum magnesium concentrations and urinary pH among participants consuming the supplemented water compared to those on control water. However, there were no significant changes observed in biomarkers related to bone metabolism or serum lipids during this period .
Comparative Studies
A comparative study evaluated the buffering capacities of magnesium hydroxide versus sodium bicarbonate formulations. Results indicated that magnesium hydroxide exhibited superior buffering stability and safety when administered orally compared to sodium bicarbonate . This highlights the potential advantages of magnesium-based compounds in clinical applications.
Data Table: Summary of Biological Activities
Biological Activity | Mechanism | Health Implications |
---|---|---|
Enzyme Activation | Cofactor for over 300 enzymes | Enhances metabolic processes |
Muscle Function | Regulates electrical impulses | Supports muscle contraction and relaxation |
Bone Health | Influences calcium metabolism | Prevents osteoporosis |
Cardiovascular Support | Regulates blood pressure | Reduces cardiovascular disease risk |
Kidney Stone Prevention | Increases calcium solubility in urine | Reduces risk of kidney stone formation |
Lung Function | Aids in muscle relaxation | Potentially improves respiratory function |
Q & A
Q. Basic: What methodologies are recommended for synthesizing magnesium bicarbonate in laboratory settings?
This compound is synthesized via reaction pathways that avoid solid-phase instability. Common methods include:
- Carbonation of magnesium hydroxide/carbonate : Dissolving Mg(OH)₂ or MgCO₃ in carbonated water under controlled CO₂ pressure (3.5–5 atm) and temperature (<50°C) to form soluble Mg(HCO₃)₂ .
- Ion exchange : Reacting magnesium acetate with sodium bicarbonate, yielding Mg(HCO₃)₂ in aqueous solution .
Key considerations : Maintain pH <8 to prevent decomposition into MgCO₃ or Mg(OH)₂. Use real-time pH monitoring and inert gas purging to stabilize dissolved CO₂ .
Q. Basic: How does this compound's instability in solid form influence experimental design?
This compound decomposes into MgCO₃, CO₂, and H₂O under ambient conditions. Researchers must:
- Avoid isolation of solid phases : Work exclusively in aqueous solutions with pH <8 and low evaporation rates .
- Use fresh preparations : Prepare solutions immediately before experiments to minimize decomposition artifacts .
Stability test protocol : Track ionic composition via ion chromatography or titration over time to quantify decomposition rates .
Q. Basic: What analytical techniques are validated for quantifying this compound in environmental water samples?
Q. Advanced: How can experimental designs account for bicarbonate concentration gradients in stability studies?
High bicarbonate concentrations (e.g., >300 mg/L) may alter Mg(HCO₃)₂ stability via ionic strength effects. Recommended approaches:
- Controlled CO₂ partial pressure : Use sealed reactors with CO₂ gas regulation to maintain consistent bicarbonate levels .
- Factorial designs : Test interactions between pH (6–8), temperature (4–25°C), and ionic strength (0.1–0.5 M NaCl) to model decomposition kinetics .
Q. Advanced: How do multivariate statistical methods resolve hydrochemical variability in magnesium-bicarbonate-rich groundwater systems?
Cluster and factor analyses (e.g., SAS CLUSTER, ArcGIS ESDA) differentiate water types:
- Factor 1 : TDS, Mg²⁺, Ca²⁺, HCO₃⁻, SO₄²⁻ (carbonate weathering dominance) .
- Factor 2 : Na⁺, Cl⁻, temperature (saline intrusion or geothermal influence) .
Data normalization : Apply logarithmic transformations to skewed Mg²⁺/HCO₃⁻ datasets to improve geostatistical accuracy .
Q. Advanced: How to address contradictions in magnesium-bicarbonate data interpretation across studies?
Contradictions arise from differing environmental contexts (e.g., geothermal vs. freshwater systems). Mitigation strategies:
- Source apportionment : Use Piper diagrams and Brine Differentiation Plots (BDP) to distinguish natural (e.g., mineral dissolution) vs. anthropogenic sources .
- Cross-validation : Compare machine learning predictions (e.g., AHP-based WQI) with field measurements to identify systemic biases .
Q. Basic: What is this compound's role in biological acid-base regulation, and how is this modeled experimentally?
Mg(HCO₃)₂ acts as a buffer, neutralizing excess H⁺ ions in physiological systems. In vitro models:
- Buffering capacity assays : Titrate Mg(HCO₃)₂ solutions with HCl/NaOH to quantify pH stabilization ranges (optimal pH 7.2–7.4) .
- Enzyme activity studies : Measure Mg²⁺-dependent enzymatic kinetics (e.g., ATPases) under varying bicarbonate concentrations .
Q. Advanced: Can machine learning improve predictive modeling of magnesium-bicarbonate interactions in complex matrices?
Yes. For example:
- AHP-weighted WQI : Assigns weights to Mg²⁺ and HCO₃⁻ using Analytic Hierarchy Process (AHP) to predict groundwater quality .
- Random Forest models : Handle non-linear relationships between Mg²⁺, HCO₃⁻, and covariates (e.g., nitrate, TDS) in large datasets .
Q. Advanced: What statistical methods are appropriate for normalizing skewed magnesium-bicarbonate data in environmental studies?
Method | Use Case | Evidence |
---|---|---|
Logarithmic transformation | Reduces skewness in Mg²⁺/HCO₃⁻ concentrations | |
Poisson regression | Models discrete variables (e.g., microbial richness linked to Mg²⁺) | |
Mixed-model ANOVA | Analyzes time-series electrolyte data (e.g., Mg²⁺ vs. bicarbonate) |
Q. Advanced: How does ionic composition (e.g., sulfate vs. bicarbonate dominance) affect magnesium speciation?
In bicarbonate-dominated systems (>98% of samples), Mg²⁺ forms stable Mg(HCO₃)₂ complexes. In sulfate-rich systems:
- Competitive ligand effects : Sulfate (SO₄²⁻) may displace HCO₃⁻, altering Mg²⁺ bioavailability .
- Geochemical modeling : Use PHREEQC to simulate Mg speciation under varying anion ratios .
Properties
IUPAC Name |
magnesium;hydrogen carbonate | |
---|---|---|
Source | PubChem | |
URL | https://pubchem.ncbi.nlm.nih.gov | |
Description | Data deposited in or computed by PubChem | |
InChI |
InChI=1S/2CH2O3.Mg/c2*2-1(3)4;/h2*(H2,2,3,4);/q;;+2/p-2 | |
Source | PubChem | |
URL | https://pubchem.ncbi.nlm.nih.gov | |
Description | Data deposited in or computed by PubChem | |
InChI Key |
QWDJLDTYWNBUKE-UHFFFAOYSA-L | |
Source | PubChem | |
URL | https://pubchem.ncbi.nlm.nih.gov | |
Description | Data deposited in or computed by PubChem | |
Canonical SMILES |
C(=O)(O)[O-].C(=O)(O)[O-].[Mg+2] | |
Source | PubChem | |
URL | https://pubchem.ncbi.nlm.nih.gov | |
Description | Data deposited in or computed by PubChem | |
Molecular Formula |
Mg(HCO3)2, C2H2MgO6 | |
Record name | Magnesium bicarbonate | |
Source | Wikipedia | |
URL | https://en.wikipedia.org/wiki/Magnesium_bicarbonate | |
Description | Chemical information link to Wikipedia. | |
Source | PubChem | |
URL | https://pubchem.ncbi.nlm.nih.gov | |
Description | Data deposited in or computed by PubChem | |
DSSTOX Substance ID |
DTXSID1062170 | |
Record name | Carbonic acid, magnesium salt (2:1) | |
Source | EPA DSSTox | |
URL | https://comptox.epa.gov/dashboard/DTXSID1062170 | |
Description | DSSTox provides a high quality public chemistry resource for supporting improved predictive toxicology. | |
Molecular Weight |
146.34 g/mol | |
Source | PubChem | |
URL | https://pubchem.ncbi.nlm.nih.gov | |
Description | Data deposited in or computed by PubChem | |
CAS No. |
2090-64-4 | |
Record name | Magnesium bicarbonate | |
Source | ChemIDplus | |
URL | https://pubchem.ncbi.nlm.nih.gov/substance/?source=chemidplus&sourceid=0002090644 | |
Description | ChemIDplus is a free, web search system that provides access to the structure and nomenclature authority files used for the identification of chemical substances cited in National Library of Medicine (NLM) databases, including the TOXNET system. | |
Record name | Carbonic acid, magnesium salt (2:1) | |
Source | EPA Chemicals under the TSCA | |
URL | https://www.epa.gov/chemicals-under-tsca | |
Description | EPA Chemicals under the Toxic Substances Control Act (TSCA) collection contains information on chemicals and their regulations under TSCA, including non-confidential content from the TSCA Chemical Substance Inventory and Chemical Data Reporting. | |
Record name | Carbonic acid, magnesium salt (2:1) | |
Source | EPA DSSTox | |
URL | https://comptox.epa.gov/dashboard/DTXSID1062170 | |
Description | DSSTox provides a high quality public chemistry resource for supporting improved predictive toxicology. | |
Record name | Magnesium bis(hydrogen carbonate) | |
Source | European Chemicals Agency (ECHA) | |
URL | https://echa.europa.eu/substance-information/-/substanceinfo/100.016.582 | |
Description | The European Chemicals Agency (ECHA) is an agency of the European Union which is the driving force among regulatory authorities in implementing the EU's groundbreaking chemicals legislation for the benefit of human health and the environment as well as for innovation and competitiveness. | |
Explanation | Use of the information, documents and data from the ECHA website is subject to the terms and conditions of this Legal Notice, and subject to other binding limitations provided for under applicable law, the information, documents and data made available on the ECHA website may be reproduced, distributed and/or used, totally or in part, for non-commercial purposes provided that ECHA is acknowledged as the source: "Source: European Chemicals Agency, http://echa.europa.eu/". Such acknowledgement must be included in each copy of the material. ECHA permits and encourages organisations and individuals to create links to the ECHA website under the following cumulative conditions: Links can only be made to webpages that provide a link to the Legal Notice page. | |
Record name | MAGNESIUM BICARBONATE | |
Source | FDA Global Substance Registration System (GSRS) | |
URL | https://gsrs.ncats.nih.gov/ginas/app/beta/substances/19E9A0647O | |
Description | The FDA Global Substance Registration System (GSRS) enables the efficient and accurate exchange of information on what substances are in regulated products. Instead of relying on names, which vary across regulatory domains, countries, and regions, the GSRS knowledge base makes it possible for substances to be defined by standardized, scientific descriptions. | |
Explanation | Unless otherwise noted, the contents of the FDA website (www.fda.gov), both text and graphics, are not copyrighted. They are in the public domain and may be republished, reprinted and otherwise used freely by anyone without the need to obtain permission from FDA. Credit to the U.S. Food and Drug Administration as the source is appreciated but not required. | |
Synthesis routes and methods I
Procedure details
Synthesis routes and methods II
Procedure details
Synthesis routes and methods III
Procedure details
Synthesis routes and methods IV
Procedure details
Synthesis routes and methods V
Procedure details
Disclaimer and Information on In-Vitro Research Products
Please be aware that all articles and product information presented on BenchChem are intended solely for informational purposes. The products available for purchase on BenchChem are specifically designed for in-vitro studies, which are conducted outside of living organisms. In-vitro studies, derived from the Latin term "in glass," involve experiments performed in controlled laboratory settings using cells or tissues. It is important to note that these products are not categorized as medicines or drugs, and they have not received approval from the FDA for the prevention, treatment, or cure of any medical condition, ailment, or disease. We must emphasize that any form of bodily introduction of these products into humans or animals is strictly prohibited by law. It is essential to adhere to these guidelines to ensure compliance with legal and ethical standards in research and experimentation.