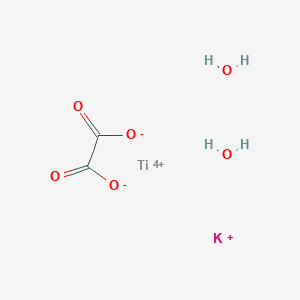
Potassium Titanium Oxalate Dihydrate
- Click on QUICK INQUIRY to receive a quote from our team of experts.
- With the quality product at a COMPETITIVE price, you can focus more on your research.
Overview
Description
Mechanism of Action
Target of Action
Potassium Titanium Oxalate Dihydrate (PTO) primarily targets aluminum alloys and hydrogen peroxide in different contexts . In the context of aluminum alloys, it is used in the micro-arc oxidation process to create a colored ceramic layer on the surface . In the context of hydrogen peroxide detection, it reacts with hydrogen peroxide to form a stable orange complex in acidic medium .
Mode of Action
In the micro-arc oxidation process, PTO is added to a sodium hexametaphosphate electrolyte system. The resulting solution is used to create a colored ceramic layer on the surface of aluminum alloys . The color of the ceramic layer is primarily due to the combined action of Al2O3 and TiO2 .
In the detection of hydrogen peroxide, PTO reacts with hydrogen peroxide in an acidic medium to form a stable orange complex. This reaction is used to measure the concentration of hydrogen peroxide in Fenton advanced oxidation systems .
Biochemical Pathways
It is known that the compound plays a role in the micro-arc oxidation process, which involves the formation of a ceramic layer on the surface of aluminum alloys . This process likely involves a number of complex chemical reactions.
Pharmacokinetics
It is known that the compound is soluble in water , which suggests that it could be readily absorbed and distributed in aqueous environments. The compound’s sensitivity to air, heat, and humidity may also affect its bioavailability.
Result of Action
The primary result of PTO’s action is the formation of a colored ceramic layer on the surface of aluminum alloys . This layer is formed as a result of the micro-arc oxidation process, and its color is primarily due to the combined action of Al2O3 and TiO2 . In the context of hydrogen peroxide detection, the reaction of PTO with hydrogen peroxide results in the formation of a stable orange complex .
Action Environment
The action of PTO is influenced by several environmental factors. For instance, the compound is sensitive to air, heat, and humidity , which can affect its stability and efficacy. In the micro-arc oxidation process, the concentration of PTO in the electrolyte solution, as well as the pulse number, pulse width, and current density, can affect the quality and color of the resulting ceramic layer .
Biochemical Analysis
Molecular Mechanism
The molecular mechanism of action of Potassium Titanium Oxalate Dihydrate is not well-defined. It’s known that it undergoes thermal dehydration in a single step through a practically irreversible process
Temporal Effects in Laboratory Settings
In laboratory settings, the thermal dehydration reaction of this compound has been studied using thermogravimetry (TG), differential thermal analysis (DTA), and differential scanning calorimetry (DSC) in a nitrogen atmosphere at different heating rates . The complexity of the dehydration of this compound is illustrated by the dependence of the activation energy on the extent of conversion .
Preparation Methods
Synthetic Routes and Reaction Conditions: Potassium Titanium Oxalate Dihydrate can be synthesized by reacting potassium oxalate with titanium oxalate in an aqueous solution. The reaction typically involves mixing equimolar amounts of potassium oxalate and titanium oxalate in water, followed by crystallization to obtain the dihydrate form .
Industrial Production Methods: In industrial settings, the production of this compound involves the controlled reaction of potassium oxalate and titanium oxalate under specific temperature and pH conditions. The resulting solution is then subjected to crystallization and drying processes to yield the dihydrate form .
Chemical Reactions Analysis
Types of Reactions: Potassium Titanium Oxalate Dihydrate undergoes various chemical reactions, including:
Oxidation: It can act as an oxidizing agent in certain reactions.
Reduction: It can be reduced under specific conditions to form titanium dioxide.
Substitution: It can participate in substitution reactions where the oxalate ligands are replaced by other ligands.
Common Reagents and Conditions:
Oxidation: Reactions typically involve oxidizing agents such as hydrogen peroxide.
Reduction: Reducing agents like sodium borohydride can be used.
Substitution: Ligand exchange reactions can be carried out using various ligands under controlled pH and temperature conditions.
Major Products:
Oxidation: Titanium dioxide (TiO2)
Reduction: Titanium dioxide (TiO2)
Substitution: Various titanium complexes depending on the ligands used.
Scientific Research Applications
Potassium Titanium Oxalate Dihydrate has a wide range of applications in scientific research:
Comparison with Similar Compounds
Titanium Oxalate: Similar in structure but lacks the potassium component.
Potassium Oxalate: Contains potassium but lacks the titanium component.
Titanium Dioxide: A common product formed from the reduction of Potassium Titanium Oxalate Dihydrate.
Uniqueness: this compound is unique due to its dual role as both a titanium and potassium source. This dual functionality allows it to participate in a wider range of chemical reactions and applications compared to its individual components .
Properties
IUPAC Name |
potassium;oxalate;titanium(4+);dihydrate |
Source
|
---|---|---|
Source | PubChem | |
URL | https://pubchem.ncbi.nlm.nih.gov | |
Description | Data deposited in or computed by PubChem | |
InChI |
InChI=1S/C2H2O4.K.2H2O.Ti/c3-1(4)2(5)6;;;;/h(H,3,4)(H,5,6);;2*1H2;/q;+1;;;+4/p-2 |
Source
|
Source | PubChem | |
URL | https://pubchem.ncbi.nlm.nih.gov | |
Description | Data deposited in or computed by PubChem | |
InChI Key |
MYYUWUVATCWCES-UHFFFAOYSA-L |
Source
|
Source | PubChem | |
URL | https://pubchem.ncbi.nlm.nih.gov | |
Description | Data deposited in or computed by PubChem | |
Canonical SMILES |
C(=O)(C(=O)[O-])[O-].O.O.[K+].[Ti+4] |
Source
|
Source | PubChem | |
URL | https://pubchem.ncbi.nlm.nih.gov | |
Description | Data deposited in or computed by PubChem | |
Molecular Formula |
C2H4KO6Ti+3 |
Source
|
Source | PubChem | |
URL | https://pubchem.ncbi.nlm.nih.gov | |
Description | Data deposited in or computed by PubChem | |
DSSTOX Substance ID |
DTXSID00747908 |
Source
|
Record name | potassium;oxalate;titanium(4+);dihydrate | |
Source | EPA DSSTox | |
URL | https://comptox.epa.gov/dashboard/DTXSID00747908 | |
Description | DSSTox provides a high quality public chemistry resource for supporting improved predictive toxicology. | |
Molecular Weight |
211.01 g/mol |
Source
|
Source | PubChem | |
URL | https://pubchem.ncbi.nlm.nih.gov | |
Description | Data deposited in or computed by PubChem | |
CAS No. |
14402-67-6 |
Source
|
Record name | potassium;oxalate;titanium(4+);dihydrate | |
Source | EPA DSSTox | |
URL | https://comptox.epa.gov/dashboard/DTXSID00747908 | |
Description | DSSTox provides a high quality public chemistry resource for supporting improved predictive toxicology. | |
Q1: What is the role of Potassium Titanium Oxalate Dihydrate in the synthesis of Barium Strontium Titanate (BaSrTiO3)?
A1: this compound serves as a precursor material for the Titanium source in the synthesis of BaSrTiO3. In the research, an oxalate co-precipitation method was employed. [] This method involves reacting Barium Chloride Dihydrate and this compound in a controlled manner to obtain a precipitate containing both Barium and Titanium. [] This precipitate is then calcined to obtain the final BaSrTiO3 powder.
Q2: Are there alternative methods for synthesizing BaSrTiO3 that don't use this compound?
A2: Yes, while the provided research uses this compound and an oxalate co-precipitation method, other methods exist. The second abstract mentions a hydrothermal process to synthesize Zinc Titanate nanoparticles. [] Although not directly stated for BaSrTiO3, this suggests that hydrothermal synthesis could be a potential alternative route. Further research would be needed to explore the efficacy of such alternative methods for BaSrTiO3 specifically.
Disclaimer and Information on In-Vitro Research Products
Please be aware that all articles and product information presented on BenchChem are intended solely for informational purposes. The products available for purchase on BenchChem are specifically designed for in-vitro studies, which are conducted outside of living organisms. In-vitro studies, derived from the Latin term "in glass," involve experiments performed in controlled laboratory settings using cells or tissues. It is important to note that these products are not categorized as medicines or drugs, and they have not received approval from the FDA for the prevention, treatment, or cure of any medical condition, ailment, or disease. We must emphasize that any form of bodily introduction of these products into humans or animals is strictly prohibited by law. It is essential to adhere to these guidelines to ensure compliance with legal and ethical standards in research and experimentation.